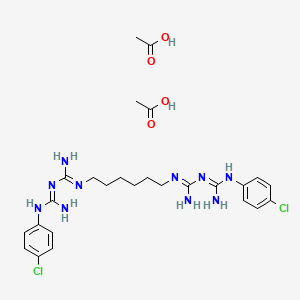
Chlorhexidine diacetate
Overview
Description
Chlorhexidine diacetate is a disinfectant and antiseptic compound with the molecular formula C26H38Cl2N10O4. It is widely used for skin disinfection before surgery, cleaning wounds, preventing dental plaque, and treating yeast infections of the mouth. It is also used to disinfect surgical instruments and to keep urinary catheters from blocking .
Mechanism of Action
Target of Action
Chlorhexidine diacetate, also known as Chlorhexidine acetate, is a broad-spectrum antimicrobial agent . It is effective against a wide range of pathogens including Gram-positive bacteria, Gram-negative bacteria, yeasts, and viruses . The primary targets of this compound are the bacterial cell membranes .
Mode of Action
This compound acts by binding to the negatively-charged sites on the bacterial cell wall due to its positively-charged molecule . This destabilizes the cell wall and interferes with osmosis . In low concentrations, it affects the integrity of the cell wall, and in high concentrations, it causes the cytoplasm to congeal or solidify .
Biochemical Pathways
The antibacterial activity of this compound is due to the elevated reactive oxygen species (ROS) production and higher lipid peroxidation . These biochemical changes result in membrane damage and alteration in the membrane proteins, phospholipids, carbohydrates, nucleic acids .
Pharmacokinetics
It binds to the proteins present in human tissues such as skin and mucous membranes . Protein-bound Chlorhexidine releases slowly, leading to prolonged activity .
Result of Action
Upon application in vitro, this compound can kill nearly 100% of Gram-positive and Gram-negative bacteria within 30 seconds . The antibacterial activity of this compound results in decreased bacterial growth, colony-forming unit (CFU) count, and cell viability .
Action Environment
From an environmental perspective, the by-products that Chlorhexidine degrades into are reported to be more toxic than Chlorhexidine itself . Further, Chlorhexidine can accumulate in the bodies of aquatic creatures and thus will increase the long-term exposure toxicity effects . It is also reported to be very toxic to aquatic organisms and may cause long-term adverse effects in the aquatic environment .
Biochemical Analysis
Biochemical Properties
Chlorhexidine diacetate plays a significant role in biochemical reactions by interacting with various enzymes, proteins, and other biomolecules. It is a cationic bis-biguanide that binds to negatively charged sites on microbial cell walls, leading to cell membrane disruption and leakage of intracellular contents . This interaction is primarily electrostatic, allowing this compound to destabilize the cell wall and interfere with osmosis . Additionally, it has been shown to bind to proteins in human tissues, such as skin and mucous membranes, leading to prolonged antimicrobial activity .
Cellular Effects
This compound exerts profound effects on various types of cells and cellular processes. It disrupts cell function by binding to the cell membrane and causing leakage of intracellular materials . This disruption affects cell signaling pathways, gene expression, and cellular metabolism. For instance, in bacterial cells, this compound causes the precipitation of cytoplasmic components, leading to cell death . In eukaryotic cells, it can cause morphological changes and impact cellular processes such as protein synthesis and energy production .
Molecular Mechanism
The molecular mechanism of this compound involves its interaction with microbial cell membranes. As a positively charged molecule, it binds to negatively charged phosphate groups on the cell surface, disrupting the integrity of the cell wall and allowing this compound to enter the cell . Once inside, it precipitates cytoplasmic components, leading to cell death . At lower concentrations, this compound exhibits bacteriostatic effects by causing leakage of intracellular substances, while at higher concentrations, it is bactericidal and causes cytoplasmic precipitation .
Temporal Effects in Laboratory Settings
In laboratory settings, the effects of this compound change over time. It is most stable within a pH range of 5 to 8, with hydrolysis occurring at extreme pH levels . Over time, this compound can degrade, especially when exposed to high temperatures or alkaline conditions . Long-term studies have shown that this compound maintains its antimicrobial activity over extended periods, although its efficacy may decrease due to degradation .
Dosage Effects in Animal Models
The effects of this compound vary with different dosages in animal models. At lower concentrations, it is effective in reducing microbial load without causing significant adverse effects . At higher doses, this compound can cause toxic effects, such as liver necrosis and bronchopneumonia in dogs . These adverse effects highlight the importance of using appropriate dosages to balance efficacy and safety.
Metabolic Pathways
This compound is known to bind to albumin in both serum and saliva, although the extent of this binding is unclear . Due to its poor absorption in the gastrointestinal tract, it is unlikely to undergo significant metabolic conversion . This limited metabolism suggests that this compound primarily exerts its effects through direct interactions with microbial cells rather than through metabolic pathways.
Transport and Distribution
Within cells and tissues, this compound is transported and distributed by binding to proteins and other biomolecules . Its positive charge allows it to interact with negatively charged sites on cell membranes, facilitating its localization and accumulation in target areas . This binding also contributes to its prolonged antimicrobial activity, as it is slowly released from bound sites over time .
Subcellular Localization
This compound localizes to specific subcellular compartments, where it exerts its antimicrobial effects. It primarily targets the cell membrane and cytoplasm, causing structural damage and leakage of intracellular contents . In bacterial cells, this compound can cause extensive lysis and loss of cytoplasmic material . In eukaryotic cells, it can induce morphological changes and impact cellular processes by interacting with membrane lipids and proteins .
Preparation Methods
Synthetic Routes and Reaction Conditions
Chlorhexidine diacetate can be synthesized by reacting hexamethylene-dicyano guanidine with p-chlorobenzoic acid amide hydrochloride in the presence of a solvent like ethylene glycol ether. The reaction is carried out under reflux conditions at 165°C for 3 hours. The resulting reaction mixture is then filtered to obtain a white solid, which is further purified to yield this compound .
Industrial Production Methods
In industrial settings, this compound is produced by similar synthetic routes but on a larger scale. The process involves precise control of reaction conditions and purification steps to ensure high yield and purity of the final product .
Chemical Reactions Analysis
Types of Reactions
Chlorhexidine diacetate undergoes various chemical reactions, including:
Oxidation: this compound can be oxidized under specific conditions, leading to the formation of different oxidation products.
Reduction: The compound can be reduced to form different reduced species.
Substitution: This compound can undergo substitution reactions, where one or more of its functional groups are replaced by other groups.
Common Reagents and Conditions
Oxidation: Common oxidizing agents such as hydrogen peroxide or potassium permanganate can be used.
Reduction: Reducing agents like sodium borohydride or lithium aluminum hydride are typically employed.
Substitution: Various nucleophiles or electrophiles can be used depending on the desired substitution.
Major Products Formed
The major products formed from these reactions depend on the specific reagents and conditions used. For example, oxidation may yield different oxidized derivatives, while reduction can produce various reduced forms of this compound .
Scientific Research Applications
Chlorhexidine diacetate has a wide range of scientific research applications:
Chemistry: It is used as a disinfectant and preservative in various chemical formulations.
Biology: this compound is employed in microbiological studies to inhibit the growth of bacteria and fungi.
Medicine: It is extensively used in medical settings for skin disinfection, wound cleaning, and as an antiseptic in dental care.
Industry: The compound is used in the production of antiseptic products, including mouthwashes, disinfectants, and preservatives .
Comparison with Similar Compounds
Chlorhexidine diacetate is often compared with other chlorhexidine salts, such as chlorhexidine digluconate and chlorhexidine dihydrochloride. While all these compounds share similar antimicrobial properties, this compound is unique in its specific applications and effectiveness in certain formulations. For example, chlorhexidine digluconate is more commonly used in dental care products, while this compound is preferred in certain antiseptic dressings .
List of Similar Compounds
- Chlorhexidine digluconate
- Chlorhexidine dihydrochloride
- Chlorhexidine free base
Properties
IUPAC Name |
acetic acid;(1E)-2-[6-[[amino-[(E)-[amino-(4-chloroanilino)methylidene]amino]methylidene]amino]hexyl]-1-[amino-(4-chloroanilino)methylidene]guanidine | |
---|---|---|
Source | PubChem | |
URL | https://pubchem.ncbi.nlm.nih.gov | |
Description | Data deposited in or computed by PubChem | |
InChI |
InChI=1S/C22H30Cl2N10.2C2H4O2/c23-15-5-9-17(10-6-15)31-21(27)33-19(25)29-13-3-1-2-4-14-30-20(26)34-22(28)32-18-11-7-16(24)8-12-18;2*1-2(3)4/h5-12H,1-4,13-14H2,(H5,25,27,29,31,33)(H5,26,28,30,32,34);2*1H3,(H,3,4) | |
Source | PubChem | |
URL | https://pubchem.ncbi.nlm.nih.gov | |
Description | Data deposited in or computed by PubChem | |
InChI Key |
WDRFFJWBUDTUCA-UHFFFAOYSA-N | |
Source | PubChem | |
URL | https://pubchem.ncbi.nlm.nih.gov | |
Description | Data deposited in or computed by PubChem | |
Canonical SMILES |
CC(=O)O.CC(=O)O.C1=CC(=CC=C1NC(=NC(=NCCCCCCN=C(N)N=C(N)NC2=CC=C(C=C2)Cl)N)N)Cl | |
Source | PubChem | |
URL | https://pubchem.ncbi.nlm.nih.gov | |
Description | Data deposited in or computed by PubChem | |
Isomeric SMILES |
CC(=O)O.CC(=O)O.C1=CC(=CC=C1N/C(=N/C(=NCCCCCCN=C(/N=C(/NC2=CC=C(C=C2)Cl)\N)N)N)/N)Cl | |
Source | PubChem | |
URL | https://pubchem.ncbi.nlm.nih.gov | |
Description | Data deposited in or computed by PubChem | |
Molecular Formula |
C26H38Cl2N10O4 | |
Source | PubChem | |
URL | https://pubchem.ncbi.nlm.nih.gov | |
Description | Data deposited in or computed by PubChem | |
DSSTOX Substance ID |
DTXSID7032345 | |
Record name | Chlorhexidine diacetate | |
Source | EPA DSSTox | |
URL | https://comptox.epa.gov/dashboard/DTXSID7032345 | |
Description | DSSTox provides a high quality public chemistry resource for supporting improved predictive toxicology. | |
Molecular Weight |
625.5 g/mol | |
Source | PubChem | |
URL | https://pubchem.ncbi.nlm.nih.gov | |
Description | Data deposited in or computed by PubChem | |
Physical Description |
White odorless solid; [Reference #1] White powder; [Aldrich MSDS] | |
Record name | Chlorhexidine acetate | |
Source | Haz-Map, Information on Hazardous Chemicals and Occupational Diseases | |
URL | https://haz-map.com/Agents/9439 | |
Description | Haz-Map® is an occupational health database designed for health and safety professionals and for consumers seeking information about the adverse effects of workplace exposures to chemical and biological agents. | |
Explanation | Copyright (c) 2022 Haz-Map(R). All rights reserved. Unless otherwise indicated, all materials from Haz-Map are copyrighted by Haz-Map(R). No part of these materials, either text or image may be used for any purpose other than for personal use. Therefore, reproduction, modification, storage in a retrieval system or retransmission, in any form or by any means, electronic, mechanical or otherwise, for reasons other than personal use, is strictly prohibited without prior written permission. | |
CAS No. |
56-95-1 | |
Record name | Chlorhexidine acetate [USP] | |
Source | ChemIDplus | |
URL | https://pubchem.ncbi.nlm.nih.gov/substance/?source=chemidplus&sourceid=0000056951 | |
Description | ChemIDplus is a free, web search system that provides access to the structure and nomenclature authority files used for the identification of chemical substances cited in National Library of Medicine (NLM) databases, including the TOXNET system. | |
Record name | Chlorhexidine diacetate | |
Source | EPA DSSTox | |
URL | https://comptox.epa.gov/dashboard/DTXSID7032345 | |
Description | DSSTox provides a high quality public chemistry resource for supporting improved predictive toxicology. | |
Record name | Chlorhexidine di(acetate) | |
Source | European Chemicals Agency (ECHA) | |
URL | https://echa.europa.eu/substance-information/-/substanceinfo/100.000.275 | |
Description | The European Chemicals Agency (ECHA) is an agency of the European Union which is the driving force among regulatory authorities in implementing the EU's groundbreaking chemicals legislation for the benefit of human health and the environment as well as for innovation and competitiveness. | |
Explanation | Use of the information, documents and data from the ECHA website is subject to the terms and conditions of this Legal Notice, and subject to other binding limitations provided for under applicable law, the information, documents and data made available on the ECHA website may be reproduced, distributed and/or used, totally or in part, for non-commercial purposes provided that ECHA is acknowledged as the source: "Source: European Chemicals Agency, http://echa.europa.eu/". Such acknowledgement must be included in each copy of the material. ECHA permits and encourages organisations and individuals to create links to the ECHA website under the following cumulative conditions: Links can only be made to webpages that provide a link to the Legal Notice page. | |
Record name | CHLORHEXIDINE ACETATE | |
Source | FDA Global Substance Registration System (GSRS) | |
URL | https://gsrs.ncats.nih.gov/ginas/app/beta/substances/5908ZUF22Y | |
Description | The FDA Global Substance Registration System (GSRS) enables the efficient and accurate exchange of information on what substances are in regulated products. Instead of relying on names, which vary across regulatory domains, countries, and regions, the GSRS knowledge base makes it possible for substances to be defined by standardized, scientific descriptions. | |
Explanation | Unless otherwise noted, the contents of the FDA website (www.fda.gov), both text and graphics, are not copyrighted. They are in the public domain and may be republished, reprinted and otherwise used freely by anyone without the need to obtain permission from FDA. Credit to the U.S. Food and Drug Administration as the source is appreciated but not required. | |
Q1: What is the primary mechanism of action of Chlorhexidine acetate against bacteria?
A1: Chlorhexidine acetate exerts its bactericidal effect primarily by disrupting the integrity and function of the bacterial cell membrane. [] This disruption leads to leakage of intracellular components and ultimately cell death.
Q2: Is there evidence suggesting other mechanisms contributing to Chlorhexidine acetate's antimicrobial activity?
A2: Yes, research suggests that active efflux pumps play a significant role in Chlorhexidine acetate resistance in bacteria like Klebsiella pneumoniae. [] This implies that efflux pump inhibition could potentially enhance Chlorhexidine acetate's efficacy.
Q3: Does Chlorhexidine acetate demonstrate any residual antimicrobial activity?
A3: Yes, studies have shown that Chlorhexidine acetate at a concentration of 0.05% exhibits residual bactericidal activity for up to 6 hours after a single irrigation in canine wound models. []
Q4: What is the molecular formula and weight of Chlorhexidine acetate?
A4: While this specific information is not directly provided in the provided research excerpts, it is widely known that Chlorhexidine diacetate (another name for Chlorhexidine acetate) possesses the molecular formula C22H30Cl2N10 · 2C2H4O2 and a molecular weight of 597.7 g/mol.
Q5: Is there any spectroscopic data available for Chlorhexidine acetate in these studies?
A6: Yes, several studies utilize ultraviolet (UV) spectrophotometry for the quantification of Chlorhexidine acetate. A common wavelength for detection is 254 nm. [] Other wavelengths like 259 nm [] and 260 nm [] are also employed depending on the specific analytical method.
Q6: How does the presence of alcohol impact the quantification of Chlorhexidine acetate using HPLC?
A7: The use of alcohol as a solvent can affect the accurate measurement of Chlorhexidine acetate content using HPLC. Research showed that increasing concentrations of ethanol, n-propanol, and isopropanol led to higher variance rates in Chlorhexidine acetate content determination. []
Q7: Can Chlorhexidine acetate be incorporated into provisional cements without compromising their properties?
A8: Research indicates that incorporating this compound at 7.5% w/w into provisional cements does not negatively impact their tensile strength, even after prolonged aging in saline solution. [] This highlights its potential for developing antimicrobial dental materials.
Q8: What is the impact of incorporating Chlorhexidine on the fluoride-releasing properties of glass ionomer cements (GICs)?
A9: Studies show that the addition of this compound or digluconate to GICs may lead to a decrease in cumulative fluoride release compared to the unmodified GIC. [] This effect is attributed to potential interactions between the cationic molecules of Chlorhexidine and fluoride ions.
Q9: Are there formulation strategies to enhance the sustained release of Chlorhexidine acetate?
A10: Yes, research highlights the development of a W/O/W multiple emulsion containing Chlorhexidine acetate, designed for prolonged release. This emulsion demonstrated stable release over 13 days and exhibited promising antibacterial effects against Porphyromonas gingivalis in vitro. []
Q10: What are the identified mechanisms of resistance to Chlorhexidine acetate in bacteria?
A11: Research primarily points to active efflux as a major mechanism of resistance to Chlorhexidine acetate, particularly in Klebsiella pneumoniae isolates. [] This highlights the potential of efflux pump inhibitors as adjunctive therapy to combat resistance.
Q11: Does this compound induce any adverse effects when used as a joint lavage fluid?
A12: Studies using a 0.05% w/v this compound solution for stifle lavage in healthy dogs revealed a more intense synovitis compared to lactated Ringer's solution. [] This finding suggests potential inflammatory effects on synovial membranes, warranting further investigation into the duration and underlying mechanisms.
Q12: What are some analytical techniques employed for the quantification of Chlorhexidine acetate?
A12: Several techniques are employed for Chlorhexidine acetate quantification, including:
- Ultraviolet (UV) spectrophotometry: Utilized for direct measurement of Chlorhexidine acetate absorbance at specific wavelengths. [, , , ]
- High-performance liquid chromatography (HPLC): Employed for separating and quantifying Chlorhexidine acetate in complex mixtures, offering high sensitivity and accuracy. [, , , , , , ]
- Resonance Rayleigh scattering (RRS) method: This method takes advantage of the enhanced scattering intensity observed when Chlorhexidine acetate interacts with specific reagents. [, ]
Q13: Have alternative analytical methods been explored for Chlorhexidine acetate determination?
A14: Yes, besides conventional techniques like HPLC and UV spectrophotometry, researchers have explored the use of charge transfer reactions for Chlorhexidine acetate quantification. [, ] This method involves reacting Chlorhexidine acetate with specific reagents to form charge transfer complexes detectable using spectrophotometry.
Disclaimer and Information on In-Vitro Research Products
Please be aware that all articles and product information presented on BenchChem are intended solely for informational purposes. The products available for purchase on BenchChem are specifically designed for in-vitro studies, which are conducted outside of living organisms. In-vitro studies, derived from the Latin term "in glass," involve experiments performed in controlled laboratory settings using cells or tissues. It is important to note that these products are not categorized as medicines or drugs, and they have not received approval from the FDA for the prevention, treatment, or cure of any medical condition, ailment, or disease. We must emphasize that any form of bodily introduction of these products into humans or animals is strictly prohibited by law. It is essential to adhere to these guidelines to ensure compliance with legal and ethical standards in research and experimentation.