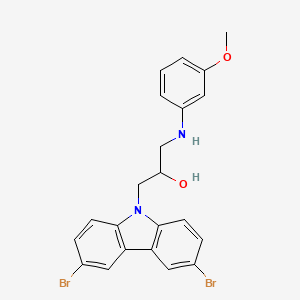
P7C3-OMe
- Click on QUICK INQUIRY to receive a quote from our team of experts.
- With the quality product at a COMPETITIVE price, you can focus more on your research.
Overview
Description
Preparation Methods
The synthesis of P7C3-OMe involves several steps:
Synthesis of 3,6-dibromo-9-(oxiran-2-ylmethyl)-9H-carbazole: This is achieved by adding powdered potassium hydroxide to a solution of 3,6-dibromocarbazole in dimethylformamide at ambient temperature. Epibromohydrin is then added, and the reaction is stirred overnight.
Formation of this compound: The intermediate product is reacted with m-anisidine in the presence of bismuth(III) chloride in cyclohexane at reflux temperature.
Chemical Reactions Analysis
P7C3-OMe undergoes various chemical reactions, including:
Oxidation: It can be oxidized under specific conditions, leading to the formation of different oxidation products.
Reduction: Reduction reactions can be performed to modify the functional groups present in the compound.
Substitution: The compound can undergo substitution reactions, particularly at the bromine atoms, to form various derivatives.
Common reagents used in these reactions include oxidizing agents like potassium permanganate, reducing agents like sodium borohydride, and nucleophiles for substitution reactions. The major products formed depend on the specific reaction conditions and reagents used.
Scientific Research Applications
P7C3-OMe has a wide range of scientific research applications:
Neuroprotection: It has been shown to protect neurons from apoptosis, making it a potential treatment for neurodegenerative diseases.
Neurogenesis: The compound promotes the formation of new neurons, which is beneficial for conditions like Alzheimer’s disease.
Cancer Research: This compound has been found to regulate aerobic glycolysis in glioma cells by targeting phosphoglycerate kinase 1, inhibiting the malignant growth of glioma.
Traumatic Brain Injury: It has been applied in the treatment of traumatic brain injury, showing efficacy in mitigating disease progression and reversing chronic neurodegeneration.
Mechanism of Action
The mechanism of action of P7C3-OMe involves the activation of nicotinamide phosphoribosyltransferase, the rate-limiting enzyme responsible for the transformation of nicotinamide into nicotinamide adenine dinucleotide. By activating this enzyme, this compound increases intracellular levels of nicotinamide adenine dinucleotide, which is crucial for cellular energy metabolism and neuroprotection .
Comparison with Similar Compounds
P7C3-OMe is part of a series of compounds with neuroprotective properties. Similar compounds include:
P7C3: The parent compound with similar neuroprotective effects.
P7C3-A20: A more potent fluorinated analogue of P7C3.
P7C3-S243: Another derivative with enhanced pharmacological functions.
This compound is unique due to its methoxy substitution, which enhances its neuroprotective and proneurogenic activities compared to its analogues .
Properties
Molecular Formula |
C22H20Br2N2O2 |
---|---|
Molecular Weight |
504.2 g/mol |
IUPAC Name |
1-(3,6-dibromocarbazol-9-yl)-3-(3-methoxyanilino)propan-2-ol |
InChI |
InChI=1S/C22H20Br2N2O2/c1-28-18-4-2-3-16(11-18)25-12-17(27)13-26-21-7-5-14(23)9-19(21)20-10-15(24)6-8-22(20)26/h2-11,17,25,27H,12-13H2,1H3 |
InChI Key |
LEICNUMXFWNCSJ-UHFFFAOYSA-N |
SMILES |
COC1=CC=CC(=C1)NCC(CN2C3=C(C=C(C=C3)Br)C4=C2C=CC(=C4)Br)O |
Canonical SMILES |
COC1=CC=CC(=C1)NCC(CN2C3=C(C=C(C=C3)Br)C4=C2C=CC(=C4)Br)O |
solubility |
not available |
Origin of Product |
United States |
Disclaimer and Information on In-Vitro Research Products
Please be aware that all articles and product information presented on BenchChem are intended solely for informational purposes. The products available for purchase on BenchChem are specifically designed for in-vitro studies, which are conducted outside of living organisms. In-vitro studies, derived from the Latin term "in glass," involve experiments performed in controlled laboratory settings using cells or tissues. It is important to note that these products are not categorized as medicines or drugs, and they have not received approval from the FDA for the prevention, treatment, or cure of any medical condition, ailment, or disease. We must emphasize that any form of bodily introduction of these products into humans or animals is strictly prohibited by law. It is essential to adhere to these guidelines to ensure compliance with legal and ethical standards in research and experimentation.