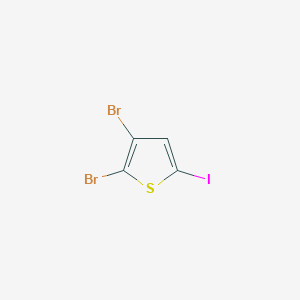
2,3-Dibromo-5-iodothiophene
- Click on QUICK INQUIRY to receive a quote from our team of experts.
- With the quality product at a COMPETITIVE price, you can focus more on your research.
Overview
Description
2,3-Dibromo-5-iodothiophene is a halogenated thiophene derivative, characterized by the presence of two bromine atoms and one iodine atom attached to the thiophene ring Thiophene is a five-membered aromatic heterocycle containing sulfur
Preparation Methods
Synthetic Routes and Reaction Conditions: The synthesis of 2,3-Dibromo-5-iodothiophene typically involves the halogenation of thiophene derivatives. One common method is the bromination of 5-iodothiophene using bromine in the presence of a catalyst such as iron or aluminum chloride. The reaction is carried out under controlled conditions to ensure selective bromination at the 2 and 3 positions.
Industrial Production Methods: Industrial production of this compound follows similar synthetic routes but on a larger scale. The process involves the use of large reactors and precise control of reaction parameters to achieve high yields and purity. The use of continuous flow reactors and automated systems can enhance the efficiency and scalability of the production process.
Chemical Reactions Analysis
Types of Reactions: 2,3-Dibromo-5-iodothiophene undergoes various chemical reactions, including:
Substitution Reactions: The bromine and iodine atoms can be replaced by other functional groups through nucleophilic or electrophilic substitution reactions.
Coupling Reactions: The compound can participate in cross-coupling reactions such as Suzuki-Miyaura and Stille couplings, forming carbon-carbon bonds with other aromatic or aliphatic compounds.
Oxidation and Reduction Reactions: The thiophene ring can undergo oxidation to form sulfoxides or sulfones, while reduction can lead to the formation of dihydrothiophenes.
Common Reagents and Conditions:
Substitution Reactions: Reagents such as sodium iodide, potassium tert-butoxide, and palladium catalysts are commonly used.
Coupling Reactions: Palladium or nickel catalysts, along with ligands like triphenylphosphine, are employed under inert atmosphere conditions.
Oxidation and Reduction Reactions: Oxidizing agents like hydrogen peroxide or m-chloroperbenzoic acid, and reducing agents like lithium aluminum hydride, are used.
Major Products:
Substitution Reactions: Products include various substituted thiophenes with functional groups like alkyl, aryl, or amino groups.
Coupling Reactions: Products are often biaryl compounds or extended conjugated systems.
Oxidation and Reduction Reactions: Products include sulfoxides, sulfones, and dihydrothiophenes.
Scientific Research Applications
2,3-Dibromo-5-iodothiophene has diverse applications in scientific research:
Chemistry: It is used as a building block in the synthesis of complex organic molecules and polymers. Its halogen atoms facilitate various coupling reactions, making it valuable in the development of new materials.
Biology: The compound is used in the synthesis of biologically active molecules, including potential pharmaceuticals and agrochemicals.
Medicine: Research into its derivatives has shown potential for developing new drugs with antimicrobial, anticancer, and anti-inflammatory properties.
Industry: It is used in the production of organic semiconductors, light-emitting diodes, and other electronic materials due to its unique electronic properties.
Mechanism of Action
The mechanism of action of 2,3-Dibromo-5-iodothiophene depends on its application. In coupling reactions, the halogen atoms act as leaving groups, allowing the formation of new carbon-carbon bonds. In biological systems, its derivatives may interact with specific molecular targets, such as enzymes or receptors, through various binding interactions, leading to the desired biological effects.
Comparison with Similar Compounds
2,3,5-Tribromothiophene: Contains three bromine atoms and exhibits similar reactivity in substitution and coupling reactions.
2,5-Dibromo-3-iodothiophene: Another isomer with different substitution patterns, affecting its reactivity and applications.
2-Bromo-3-iodothiophene: Contains one bromine and one iodine atom, used in similar coupling reactions but with different reactivity profiles.
Uniqueness: 2,3-Dibromo-5-iodothiophene is unique due to its specific substitution pattern, which provides distinct reactivity and selectivity in chemical reactions. The presence of both bromine and iodine atoms allows for versatile functionalization, making it a valuable intermediate in organic synthesis.
Properties
IUPAC Name |
2,3-dibromo-5-iodothiophene |
Source
|
---|---|---|
Details | Computed by LexiChem 2.6.6 (PubChem release 2019.06.18) | |
Source | PubChem | |
URL | https://pubchem.ncbi.nlm.nih.gov | |
Description | Data deposited in or computed by PubChem | |
InChI |
InChI=1S/C4HBr2IS/c5-2-1-3(7)8-4(2)6/h1H |
Source
|
Details | Computed by InChI 1.0.5 (PubChem release 2019.06.18) | |
Source | PubChem | |
URL | https://pubchem.ncbi.nlm.nih.gov | |
Description | Data deposited in or computed by PubChem | |
InChI Key |
GBJCHTHMOAJBOD-UHFFFAOYSA-N |
Source
|
Details | Computed by InChI 1.0.5 (PubChem release 2019.06.18) | |
Source | PubChem | |
URL | https://pubchem.ncbi.nlm.nih.gov | |
Description | Data deposited in or computed by PubChem | |
Canonical SMILES |
C1=C(SC(=C1Br)Br)I |
Source
|
Details | Computed by OEChem 2.1.5 (PubChem release 2019.06.18) | |
Source | PubChem | |
URL | https://pubchem.ncbi.nlm.nih.gov | |
Description | Data deposited in or computed by PubChem | |
Molecular Formula |
C4HBr2IS |
Source
|
Details | Computed by PubChem 2.1 (PubChem release 2019.06.18) | |
Source | PubChem | |
URL | https://pubchem.ncbi.nlm.nih.gov | |
Description | Data deposited in or computed by PubChem | |
Molecular Weight |
367.83 g/mol |
Source
|
Details | Computed by PubChem 2.1 (PubChem release 2021.05.07) | |
Source | PubChem | |
URL | https://pubchem.ncbi.nlm.nih.gov | |
Description | Data deposited in or computed by PubChem | |
Disclaimer and Information on In-Vitro Research Products
Please be aware that all articles and product information presented on BenchChem are intended solely for informational purposes. The products available for purchase on BenchChem are specifically designed for in-vitro studies, which are conducted outside of living organisms. In-vitro studies, derived from the Latin term "in glass," involve experiments performed in controlled laboratory settings using cells or tissues. It is important to note that these products are not categorized as medicines or drugs, and they have not received approval from the FDA for the prevention, treatment, or cure of any medical condition, ailment, or disease. We must emphasize that any form of bodily introduction of these products into humans or animals is strictly prohibited by law. It is essential to adhere to these guidelines to ensure compliance with legal and ethical standards in research and experimentation.