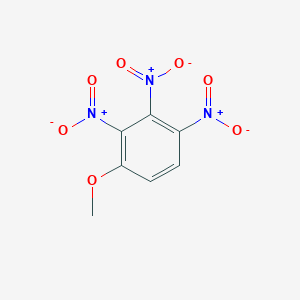
1-Methoxy-2,3,4-trinitrobenzene
- Click on QUICK INQUIRY to receive a quote from our team of experts.
- With the quality product at a COMPETITIVE price, you can focus more on your research.
Overview
Description
1-Methoxy-2,3,4-trinitrobenzene is a derivative of benzene . It contains a total of 22 bonds; 17 non-H bonds, 12 multiple bonds, 4 rotatable bonds, 6 double bonds, 6 aromatic bonds, 1 six-membered ring, 3 nitro groups (aromatic), and 1 ether (aromatic) .
Molecular Structure Analysis
The molecular structure of this compound includes a benzene ring with three nitro groups and one methoxy group attached . The nitro group, like the carboxylate anion, is a hybrid of two equivalent resonance structures .Chemical Reactions Analysis
While specific chemical reactions involving this compound are not detailed in the sources retrieved, nitro compounds in general are quite unstable in the thermodynamic sense .Physical And Chemical Properties Analysis
This compound is described as white or yellowish needle-like crystals. It is almost insoluble in water, slightly soluble in ethanol, soluble in benzene, toluene, and acetone . The nitro group contributes to the high dipole moments of nitro compounds .Scientific Research Applications
Oxygen Carriers and Molecular Binding
1-Methoxy-2,3,4-trinitrobenzene, along with other trinitrobenzene derivatives, has been studied for its capability in reversible dimerization after electrochemical reduction. This process leads to the formation of a pi-dimer that can capture molecular oxygen (O2), creating a sigma(o-o)(H)-adduct. This adduct shows potential in reversibly binding and removing dioxygen after an external redox stimulus, indicating its utility in oxygen transport and storage mechanisms (Gallardo & Guirado, 2008).
Molecular Complex Formation
Research has also delved into the formation of molecular complexes between 1,3,5-trinitrobenzene and various methoxy-substituted indoles. The study determined the association constants for these complexes by measuring chemical shifts in their nuclear magnetic resonance spectra. The findings provide insights into the structure and stability of these complexes, which are critical for understanding molecular interactions in various chemical processes (Sung & Parker, 1972).
Meisenheimer Complex Studies
The stable methoxide addition complexes of this compound and related compounds have been characterized as Meisenheimer (σ−) complexes, revealing intricate details about the addition of base in specific positions. This research helps in comprehending the structural aspects and reactivity patterns of such complexes (Caveng et al., 1967).
High Energy Materials (HEMs)
In the context of green chemistry, this compound derivatives have been synthesized using eco-friendly approaches. These compounds, characterized by spectroscopic techniques, show relevance as high energy materials, offering insights into the potential of such compounds in various applications requiring energy-dense materials (Badgujar et al., 2008).
Safety and Hazards
Mechanism of Action
Target of Action
1-Methoxy-2,3,4-trinitrobenzene is a type of nitro compound . Nitro compounds are a significant class of nitrogen derivatives, with the nitro group (−NO2) being a hybrid of two equivalent resonance structures . The primary targets of nitro compounds are typically organic molecules, where they can participate in various chemical reactions .
Mode of Action
The mode of action of this compound involves its interaction with its targets through various chemical reactions. Nitro compounds can participate in reactions such as direct substitution of hydrocarbons with nitric acid, displacement reactions with nitrite ions, and oxidation of primary amines . These reactions can result in significant changes in the target molecules .
Biochemical Pathways
The biochemical pathways affected by this compound are primarily those involving the reactions of nitro compounds. For instance, nitration of alkanes and aromatic compounds such as benzene can occur . These reactions can have downstream effects on other biochemical pathways, potentially leading to the formation of new compounds .
Pharmacokinetics
The pharmacokinetics of this compound would involve its absorption, distribution, metabolism, and excretion (ADME). Nitro compounds, in general, have lower volatility than ketones of about the same molecular weight, and their water solubility is low . These properties could impact the bioavailability of this compound.
Result of Action
The molecular and cellular effects of the action of this compound would depend on the specific reactions it undergoes with its targets. As a nitro compound, it could potentially participate in various chemical reactions leading to the formation of new compounds . The exact outcomes would depend on the specific conditions and the presence of other reactants .
Action Environment
The action, efficacy, and stability of this compound can be influenced by various environmental factors. For instance, nitration of alkanes by nitro compounds is successful only when conducted at high temperatures in the vapor phase . Furthermore, the polar character of the nitro group can influence the compound’s interactions with its environment .
properties
IUPAC Name |
1-methoxy-2,3,4-trinitrobenzene |
Source
|
---|---|---|
Details | Computed by LexiChem 2.6.6 (PubChem release 2019.06.18) | |
Source | PubChem | |
URL | https://pubchem.ncbi.nlm.nih.gov | |
Description | Data deposited in or computed by PubChem | |
InChI |
InChI=1S/C7H5N3O7/c1-17-5-3-2-4(8(11)12)6(9(13)14)7(5)10(15)16/h2-3H,1H3 |
Source
|
Details | Computed by InChI 1.0.5 (PubChem release 2019.06.18) | |
Source | PubChem | |
URL | https://pubchem.ncbi.nlm.nih.gov | |
Description | Data deposited in or computed by PubChem | |
InChI Key |
ATAUKBZXCKSYQR-UHFFFAOYSA-N |
Source
|
Details | Computed by InChI 1.0.5 (PubChem release 2019.06.18) | |
Source | PubChem | |
URL | https://pubchem.ncbi.nlm.nih.gov | |
Description | Data deposited in or computed by PubChem | |
Canonical SMILES |
COC1=C(C(=C(C=C1)[N+](=O)[O-])[N+](=O)[O-])[N+](=O)[O-] |
Source
|
Details | Computed by OEChem 2.1.5 (PubChem release 2019.06.18) | |
Source | PubChem | |
URL | https://pubchem.ncbi.nlm.nih.gov | |
Description | Data deposited in or computed by PubChem | |
Molecular Formula |
C7H5N3O7 |
Source
|
Details | Computed by PubChem 2.1 (PubChem release 2019.06.18) | |
Source | PubChem | |
URL | https://pubchem.ncbi.nlm.nih.gov | |
Description | Data deposited in or computed by PubChem | |
Molecular Weight |
243.13 g/mol |
Source
|
Details | Computed by PubChem 2.1 (PubChem release 2021.05.07) | |
Source | PubChem | |
URL | https://pubchem.ncbi.nlm.nih.gov | |
Description | Data deposited in or computed by PubChem | |
Disclaimer and Information on In-Vitro Research Products
Please be aware that all articles and product information presented on BenchChem are intended solely for informational purposes. The products available for purchase on BenchChem are specifically designed for in-vitro studies, which are conducted outside of living organisms. In-vitro studies, derived from the Latin term "in glass," involve experiments performed in controlled laboratory settings using cells or tissues. It is important to note that these products are not categorized as medicines or drugs, and they have not received approval from the FDA for the prevention, treatment, or cure of any medical condition, ailment, or disease. We must emphasize that any form of bodily introduction of these products into humans or animals is strictly prohibited by law. It is essential to adhere to these guidelines to ensure compliance with legal and ethical standards in research and experimentation.