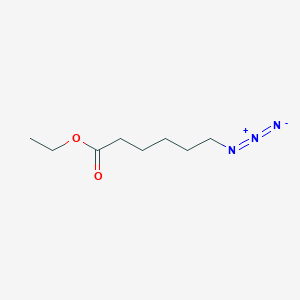
Ethyl 6-azidohexanoate
Overview
Description
Ethyl 6-azidohexanoate is an organic compound characterized by the presence of an ethyl ester and a terminal azide group connected by a six-carbon chain. Its molecular formula is C8H15N3O2, and it has a molecular weight of 185.22 g/mol . This compound is notable for its reactivity, particularly in click chemistry, where it forms stable triazole linkages with alkynes .
Preparation Methods
Synthetic Routes and Reaction Conditions: Ethyl 6-azidohexanoate can be synthesized through a multi-step process involving the conversion of 6-bromohexanoic acid to 6-azidohexanoic acid, followed by esterification. The typical synthetic route includes:
Nucleophilic Substitution: 6-bromohexanoic acid is reacted with sodium azide (NaN3) in a polar aprotic solvent like dimethyl sulfoxide (DMSO) to form 6-azidohexanoic acid.
Industrial Production Methods: While specific industrial production methods for this compound are not widely documented, the general approach involves scaling up the laboratory synthesis process. This includes optimizing reaction conditions to ensure high yield and purity, as well as implementing safety measures due to the potentially hazardous nature of azides.
Chemical Reactions Analysis
Types of Reactions: Ethyl 6-azidohexanoate undergoes various chemical reactions, including:
Click Chemistry: The azide group reacts with alkynes in the presence of a copper(I) catalyst to form 1,2,3-triazoles.
Hydrolysis: The ester group can be hydrolyzed to form 6-azidohexanoic acid under acidic or basic conditions.
Common Reagents and Conditions:
Click Chemistry: Copper(I) sulfate (CuSO4) and sodium ascorbate in a solvent like water or tert-butanol.
Hydrolysis: Aqueous hydrochloric acid (HCl) or sodium hydroxide (NaOH).
Major Products:
Click Chemistry: 1,2,3-triazoles.
Reduction: 6-aminohexanoic acid.
Hydrolysis: 6-azidohexanoic acid.
Scientific Research Applications
Ethyl 6-azidohexanoate has diverse applications in scientific research:
Chemistry: Used as a building block in the synthesis of complex molecules through click chemistry.
Biology: Employed in bioconjugation techniques to label biomolecules with fluorescent tags or other probes.
Medicine: Investigated for its potential in drug delivery systems and the development of novel therapeutics.
Industry: Utilized in the production of polymers and materials with specific functional properties.
Mechanism of Action
The primary mechanism of action for ethyl 6-azidohexanoate involves its reactivity in click chemistry. The azide group reacts with alkynes to form stable triazole linkages, which are valuable in various applications, including drug development and material science . The molecular targets and pathways involved in these reactions are primarily dictated by the nature of the alkyne and the specific conditions used in the reaction .
Comparison with Similar Compounds
6-Azidohexanoic acid: Similar structure but lacks the ethyl ester group.
Ethyl 4-azidobutanoate: Shorter carbon chain but similar functional groups.
Ethyl 8-azidooctanoate: Longer carbon chain but similar functional groups.
Uniqueness: Ethyl 6-azidohexanoate is unique due to its optimal chain length, which provides a balance between reactivity and stability. This makes it particularly useful in click chemistry and bioconjugation applications .
Properties
IUPAC Name |
ethyl 6-azidohexanoate | |
---|---|---|
Details | Computed by LexiChem 2.6.6 (PubChem release 2019.06.18) | |
Source | PubChem | |
URL | https://pubchem.ncbi.nlm.nih.gov | |
Description | Data deposited in or computed by PubChem | |
InChI |
InChI=1S/C8H15N3O2/c1-2-13-8(12)6-4-3-5-7-10-11-9/h2-7H2,1H3 | |
Details | Computed by InChI 1.0.5 (PubChem release 2019.06.18) | |
Source | PubChem | |
URL | https://pubchem.ncbi.nlm.nih.gov | |
Description | Data deposited in or computed by PubChem | |
InChI Key |
ONUGSWFFTHJOQF-UHFFFAOYSA-N | |
Details | Computed by InChI 1.0.5 (PubChem release 2019.06.18) | |
Source | PubChem | |
URL | https://pubchem.ncbi.nlm.nih.gov | |
Description | Data deposited in or computed by PubChem | |
Canonical SMILES |
CCOC(=O)CCCCCN=[N+]=[N-] | |
Details | Computed by OEChem 2.1.5 (PubChem release 2019.06.18) | |
Source | PubChem | |
URL | https://pubchem.ncbi.nlm.nih.gov | |
Description | Data deposited in or computed by PubChem | |
Molecular Formula |
C8H15N3O2 | |
Details | Computed by PubChem 2.1 (PubChem release 2019.06.18) | |
Source | PubChem | |
URL | https://pubchem.ncbi.nlm.nih.gov | |
Description | Data deposited in or computed by PubChem | |
Molecular Weight |
185.22 g/mol | |
Details | Computed by PubChem 2.1 (PubChem release 2021.05.07) | |
Source | PubChem | |
URL | https://pubchem.ncbi.nlm.nih.gov | |
Description | Data deposited in or computed by PubChem | |
Synthesis routes and methods I
Procedure details
Synthesis routes and methods II
Procedure details
Disclaimer and Information on In-Vitro Research Products
Please be aware that all articles and product information presented on BenchChem are intended solely for informational purposes. The products available for purchase on BenchChem are specifically designed for in-vitro studies, which are conducted outside of living organisms. In-vitro studies, derived from the Latin term "in glass," involve experiments performed in controlled laboratory settings using cells or tissues. It is important to note that these products are not categorized as medicines or drugs, and they have not received approval from the FDA for the prevention, treatment, or cure of any medical condition, ailment, or disease. We must emphasize that any form of bodily introduction of these products into humans or animals is strictly prohibited by law. It is essential to adhere to these guidelines to ensure compliance with legal and ethical standards in research and experimentation.