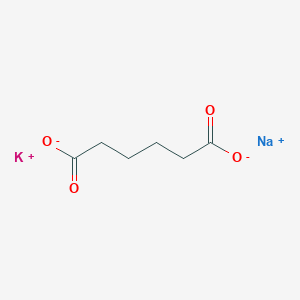
Potassium sodium adipate
Overview
Description
Inositol 1,3,4,5-Tetrakisphosphate is a member of the inositol phosphate family, which are signaling molecules playing crucial roles in various cellular processes. This compound is particularly significant due to its involvement in cellular signaling pathways, especially those related to calcium release and regulation .
Preparation Methods
Synthetic Routes and Reaction Conditions: Inositol 1,3,4,5-Tetrakisphosphate can be synthesized through a series of phosphorylation reactions. One common method involves the phosphorylation of inositol 1,4,5-trisphosphate by inositol trisphosphate kinases . The reaction conditions typically require specific enzymes and controlled environments to ensure the correct phosphorylation at the desired positions .
Industrial Production Methods: Industrial production of inositol 1,3,4,5-Tetrakisphosphate is less common due to the complexity of its synthesis. advancements in biotechnology and enzymatic synthesis have made it possible to produce this compound on a larger scale. The use of recombinant enzymes and optimized reaction conditions are key factors in industrial production .
Chemical Reactions Analysis
Types of Reactions: Inositol 1,3,4,5-Tetrakisphosphate undergoes various chemical reactions, including phosphorylation, dephosphorylation, and binding interactions with proteins . These reactions are crucial for its role as a signaling molecule.
Common Reagents and Conditions: The phosphorylation reactions typically involve the use of ATP and specific kinases, while dephosphorylation requires phosphatases . The binding interactions often occur under physiological conditions, such as in the presence of calcium ions .
Major Products Formed: The major products formed from these reactions include various inositol phosphates, such as inositol 1,4,5-trisphosphate and inositol hexakisphosphate . These products play significant roles in cellular signaling and regulation .
Scientific Research Applications
Applications in the Food Industry
Potassium sodium adipate serves several important functions in food technology:
- Buffering Agent : It helps maintain pH stability in food products, which is crucial for flavor preservation and microbial stability.
- Acidity Regulator : By regulating acidity, it enhances the taste and texture of various food items, particularly in baked goods and dairy products.
- Starch Retrogradation Inhibition : Research indicates that it can inhibit retrogradation in starches, improving the texture and shelf-life of food products.
Table 1: Key Functions of this compound in Food Applications
Function | Description |
---|---|
Buffering Agent | Maintains pH stability in food products |
Acidity Regulator | Enhances flavor and texture |
Starch Retrogradation Inhibitor | Improves texture and shelf-life of starchy foods |
Pharmaceutical Applications
In the pharmaceutical sector, this compound is employed for:
- Excipient Properties : It stabilizes active pharmaceutical ingredients (APIs) in formulations, enhancing their efficacy and shelf-life.
- pH Regulation : Similar to its role in food, it regulates pH levels in drug formulations, which can be critical for drug solubility and absorption.
Case Study: Use in Drug Formulations
A study demonstrated that incorporating this compound into a specific drug formulation improved the stability of the active ingredient under various storage conditions. The excipient's buffering capacity helped maintain the required pH level, ensuring optimal drug performance over time.
Scientific Research Applications
This compound is utilized extensively in scientific research:
- Biochemical Studies : It serves as a buffering agent in biological experiments, helping to maintain physiological pH levels during assays.
- Metabolic Pathway Research : The compound participates in metabolic pathways involving fatty acids, providing insights into energy production processes within cells .
Table 2: Research Applications of this compound
Application | Description |
---|---|
Biochemical Studies | Used to maintain pH levels during experiments |
Metabolic Pathway Research | Investigates fatty acid metabolism |
Environmental Considerations
While this compound is widely used, it is essential to consider its environmental impact. As with many chemical compounds, it can contribute to pollution if not managed properly during production and disposal. Research into biodegradable alternatives is ongoing to mitigate these effects.
Mechanism of Action
Inositol 1,3,4,5-Tetrakisphosphate exerts its effects by binding to specific protein domains, such as the pleckstrin homology domain . This binding regulates the activity of various enzymes and proteins involved in cellular signaling pathways . The compound primarily targets pathways related to calcium release and regulation, influencing processes such as cell proliferation, apoptosis, and metabolism .
Comparison with Similar Compounds
Similar Compounds:
- Inositol 1,4,5-Trisphosphate
- Inositol Hexakisphosphate
- Inositol 1,3,4,6-Tetrakisphosphate
Uniqueness: Inositol 1,3,4,5-Tetrakisphosphate is unique due to its specific phosphorylation pattern, which allows it to interact with distinct protein domains and regulate unique signaling pathways . This specificity makes it a valuable tool for studying cellular processes and developing therapeutic applications .
Biological Activity
Potassium sodium adipate, a potassium salt of adipic acid, is primarily recognized for its role in various biochemical and physiological processes. This article will explore its biological activity, mechanisms of action, and relevant research findings, including case studies and data tables to illustrate its effects.
This compound is derived from adipic acid, a dicarboxylic acid commonly used in the production of nylon and as a food additive. The chemical formula is represented as , indicating the presence of both potassium (K) and sodium (Na) ions along with adipate ions. This compound is soluble in water, where it dissociates into its constituent ions, which play significant roles in biological systems.
Biochemical Pathways:
this compound acts as a substrate for enzymes involved in fatty acid metabolism. It facilitates the conversion of adipic acid derivatives into energy through β-oxidation pathways. This metabolic process is crucial for energy production in cells, particularly in muscle tissues .
pH Regulation:
One of the key biological activities of this compound is its ability to regulate pH levels within various environments. By acting as a buffering agent, it helps maintain stability in biochemical reactions sensitive to pH fluctuations. This property is particularly beneficial in food products, enhancing taste and shelf-life .
Cellular Impact
This compound influences cellular functions by modulating cell signaling pathways and gene expression. It has been shown to affect sodium ion concentrations within cells, which can alter various cellular processes such as nutrient uptake and metabolic activity.
Dosage Effects
Research indicates that the effects of this compound vary with dosage levels. In animal models, low doses are generally well-tolerated without significant adverse effects; however, higher doses may lead to gastrointestinal disturbances or allergic reactions .
Toxicological Studies
A comprehensive review of toxicological data reveals that this compound has low acute toxicity. The oral LD50 (lethal dose for 50% of the population) in rats is approximately 5560 mg/kg body weight, indicating a relatively safe profile at normal consumption levels . Long-term studies have not demonstrated significant toxicity or genotoxic potential .
Case Studies
-
Chronic Kidney Disease (CKD) Management:
A study highlighted the implications of potassium additives, including this compound, in dietary management for CKD patients. It was found that while moderate potassium intake may provide protective cardiovascular benefits early in the disease, excessive intake could lead to hyperkalemia in advanced stages . -
Food Additive Safety:
Research evaluating food additives containing potassium showed that 37.6% of processed products analyzed contained at least one potassium additive, raising concerns among health professionals regarding their impact on health, particularly for sensitive populations like CKD patients .
Table 1: Summary of Biological Effects of this compound
Effect | Description |
---|---|
Energy Metabolism | Acts as a substrate for fatty acid metabolism |
pH Buffering | Regulates acidity in food products |
Cellular Function Modulation | Influences cell signaling and sodium ion concentration |
Toxicity Profile | Low acute toxicity; LD50 ~ 5560 mg/kg in rats |
Table 2: Dosage Effects Observed in Animal Models
Dosage Level (mg/kg) | Observed Effects |
---|---|
Low (up to 1000) | Well-tolerated; no significant adverse effects |
Moderate (1000-3000) | Mild gastrointestinal disturbances |
High (above 3000) | Potential allergic reactions |
Q & A
Basic Research Questions
Q. What are the standard laboratory synthesis methods for potassium sodium adipate, and how do reaction conditions influence yield?
this compound is synthesized via neutralization reactions between adipic acid and sodium/potassium hydroxides. A common approach involves dissolving adipic acid in a 1:1 v/v dimethyl sulfoxide (DMSO)/water solvent mixture, followed by controlled addition of sodium hydroxide and potassium hydroxide to achieve a stoichiometric ratio. Temperature (maintained at 60–80°C) and pH (adjusted to 6.5–7.5) are critical for optimizing crystallinity and yield. Post-synthesis, vacuum filtration and recrystallization in ethanol are used to purify the product .
Q. How are potassium and sodium adipates characterized for purity and structural integrity in academic research?
Researchers employ a combination of elemental analysis (to confirm Na/K ratios), Fourier-transform infrared spectroscopy (FT-IR) (to identify carboxylate stretching bands at ~1550–1600 cm⁻¹), and X-ray diffraction (XRD) (to verify crystallinity). Thermogravimetric analysis (TGA) is used to assess thermal stability, with decomposition typically observed above 250°C .
Q. What role do sodium and potassium adipates play in food science applications, and how are their effects quantified?
As acidity regulators (E356/E357), these salts modulate pH in food matrices like baked goods and beverages. Their efficacy is tested via pH titration curves and rheological studies to measure impacts on dough elasticity or emulsion stability. For example, in weaning foods, sodium adipate enhances gelling when combined with acetylated distarch adipate (E1422), evaluated using texture profile analysis (TPA) .
Q. What experimental protocols are recommended for studying adipate salts in biodegradable polymer formulations?
In polymer blends (e.g., with polybutylene adipate terephthalate, PBAT), adipate salts are incorporated via solvent casting or extrusion. Researchers assess biodegradability using soil burial tests (mass loss over 12 weeks) and enzymatic degradation assays (lipase/cutinase activity). Mechanical properties are quantified via tensile testing (ASTM D638) .
Advanced Research Questions
Q. How do ligand variations (e.g., oxalate, phenanthroline) influence the structural dimensionality of adipate-based coordination polymers?
Hydrothermal synthesis with cadmium(II) and oxalate ligands induces structural transformations from 1D chains to 3D frameworks, as shown by single-crystal XRD. The oxalate ligand bridges adjacent chains, altering coordination geometry (e.g., monoclinic C2/c to Cc space groups). Fluorescence quenching studies reveal ligand-dependent electronic transitions .
Q. What strategies resolve contradictions in spectroscopic data when analyzing adipate salt degradation byproducts?
Discrepancies between FT-IR and NMR data (e.g., unexpected carbonyl signals) are addressed through controlled hydrolysis experiments and high-resolution mass spectrometry (HR-MS) . For instance, adipate ester hydrolysis in acidic conditions generates succinic acid intermediates, identifiable via GC-MS .
Q. How do pH and counterion ratios affect the electrochemical stability of adipate salts in energy storage applications?
Cyclic voltammetry (CV) in 0.1 M KCl reveals that sodium-rich adipate formulations exhibit higher anodic stability (+1.2 V vs. Ag/AgCl) compared to potassium-dominant salts. Ionic conductivity is measured via electrochemical impedance spectroscopy (EIS), with Na⁺ showing lower charge-transfer resistance .
Q. What advanced techniques are used to probe the interaction between adipate salts and biomacromolecules (e.g., proteins, polysaccharides)?
Isothermal titration calorimetry (ITC) quantifies binding affinities between sodium adipate and bovine serum albumin (BSA), while small-angle X-ray scattering (SAXS) maps structural changes in starch-adipate complexes during gelatinization .
Q. How do adipate salts influence the nucleation kinetics of biodegradable polyesters during melt processing?
Differential scanning calorimetry (DSC) shows that potassium adipate reduces the crystallization half-time (t₁/₂) of PBAT by 30% at 5 wt% loading, acting as a nucleating agent. Polarized optical microscopy (POM) confirms spherulitic growth patterns .
Q. What computational models predict the environmental fate of adipate salts in aqueous systems?
Density functional theory (DFT) simulations model adipate degradation pathways, identifying hydroxyl radical (•OH) attack as the primary mechanism in advanced oxidation processes (AOPs). Experimental validation uses liquid chromatography-tandem mass spectrometry (LC-MS/MS) to detect hydroxylated intermediates .
Q. Methodological Notes
- Data Contradiction Analysis : Cross-validate spectroscopic and chromatographic results with replicate experiments and alternative techniques (e.g., XRD vs. SAXS for structural confirmation).
- Experimental Design : For coordination polymer studies, prioritize hydrothermal synthesis with in situ pH monitoring to stabilize target phases .
- Biodegradability Testing : Use ISO 14855 standards for compost conditions, with microbial diversity analysis via 16S rRNA sequencing to link degradation rates to microbiome shifts .
Properties
IUPAC Name |
potassium;sodium;hexanedioate | |
---|---|---|
Source | PubChem | |
URL | https://pubchem.ncbi.nlm.nih.gov | |
Description | Data deposited in or computed by PubChem | |
InChI |
InChI=1S/C6H10O4.K.Na/c7-5(8)3-1-2-4-6(9)10;;/h1-4H2,(H,7,8)(H,9,10);;/q;2*+1/p-2 | |
Source | PubChem | |
URL | https://pubchem.ncbi.nlm.nih.gov | |
Description | Data deposited in or computed by PubChem | |
InChI Key |
UAYBDPAIBGXPQE-UHFFFAOYSA-L | |
Source | PubChem | |
URL | https://pubchem.ncbi.nlm.nih.gov | |
Description | Data deposited in or computed by PubChem | |
Canonical SMILES |
C(CCC(=O)[O-])CC(=O)[O-].[Na+].[K+] | |
Source | PubChem | |
URL | https://pubchem.ncbi.nlm.nih.gov | |
Description | Data deposited in or computed by PubChem | |
Molecular Formula |
C6H8KNaO4 | |
Source | PubChem | |
URL | https://pubchem.ncbi.nlm.nih.gov | |
Description | Data deposited in or computed by PubChem | |
DSSTOX Substance ID |
DTXSID40173247 | |
Record name | Potassium sodium adipate | |
Source | EPA DSSTox | |
URL | https://comptox.epa.gov/dashboard/DTXSID40173247 | |
Description | DSSTox provides a high quality public chemistry resource for supporting improved predictive toxicology. | |
Molecular Weight |
206.21 g/mol | |
Source | PubChem | |
URL | https://pubchem.ncbi.nlm.nih.gov | |
Description | Data deposited in or computed by PubChem | |
CAS No. |
19584-53-3 | |
Record name | Potassium sodium adipate | |
Source | ChemIDplus | |
URL | https://pubchem.ncbi.nlm.nih.gov/substance/?source=chemidplus&sourceid=0019584533 | |
Description | ChemIDplus is a free, web search system that provides access to the structure and nomenclature authority files used for the identification of chemical substances cited in National Library of Medicine (NLM) databases, including the TOXNET system. | |
Record name | Potassium sodium adipate | |
Source | EPA DSSTox | |
URL | https://comptox.epa.gov/dashboard/DTXSID40173247 | |
Description | DSSTox provides a high quality public chemistry resource for supporting improved predictive toxicology. | |
Record name | Potassium sodium adipate | |
Source | European Chemicals Agency (ECHA) | |
URL | https://echa.europa.eu/substance-information/-/substanceinfo/100.039.233 | |
Description | The European Chemicals Agency (ECHA) is an agency of the European Union which is the driving force among regulatory authorities in implementing the EU's groundbreaking chemicals legislation for the benefit of human health and the environment as well as for innovation and competitiveness. | |
Explanation | Use of the information, documents and data from the ECHA website is subject to the terms and conditions of this Legal Notice, and subject to other binding limitations provided for under applicable law, the information, documents and data made available on the ECHA website may be reproduced, distributed and/or used, totally or in part, for non-commercial purposes provided that ECHA is acknowledged as the source: "Source: European Chemicals Agency, http://echa.europa.eu/". Such acknowledgement must be included in each copy of the material. ECHA permits and encourages organisations and individuals to create links to the ECHA website under the following cumulative conditions: Links can only be made to webpages that provide a link to the Legal Notice page. | |
Disclaimer and Information on In-Vitro Research Products
Please be aware that all articles and product information presented on BenchChem are intended solely for informational purposes. The products available for purchase on BenchChem are specifically designed for in-vitro studies, which are conducted outside of living organisms. In-vitro studies, derived from the Latin term "in glass," involve experiments performed in controlled laboratory settings using cells or tissues. It is important to note that these products are not categorized as medicines or drugs, and they have not received approval from the FDA for the prevention, treatment, or cure of any medical condition, ailment, or disease. We must emphasize that any form of bodily introduction of these products into humans or animals is strictly prohibited by law. It is essential to adhere to these guidelines to ensure compliance with legal and ethical standards in research and experimentation.