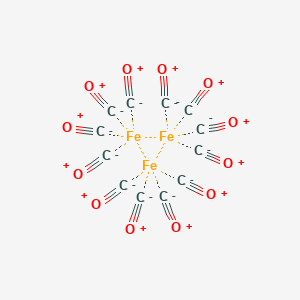
Triiron dodecacarbonyl
Overview
Description
Preparation Methods
Triiron dodecacarbonyl can be synthesized through several methods:
-
Thermolysis of Iron Pentacarbonyl: : One of the earliest methods involves the thermolysis of iron pentacarbonyl (Fe(CO)₅): [ 3 \text{Fe(CO)}_5 \rightarrow \text{Fe}3(\text{CO}){12} + 3 \text{CO} ] This reaction is typically carried out under controlled conditions to ensure the formation of this compound .
-
Reaction with Base and Subsequent Oxidation: : Another common method involves the reaction of iron pentacarbonyl with a base, followed by oxidation: [ 3 \text{Fe(CO)}_5 + (\text{C}_2\text{H}_5)_3\text{N} + \text{H}_2\text{O} \rightarrow [(\text{C}_2\text{H}_5)_3\text{NH}][\text{HFe}3(\text{CO}){11}] + 3 \text{CO} + \text{CO}_2 ] The resulting hydrido cluster is then oxidized with acid to form this compound .
Chemical Reactions Analysis
Triiron dodecacarbonyl undergoes various chemical reactions, including:
Oxidation: It can be oxidized to form different iron carbonyl complexes.
Reduction: Reduction reactions can lead to the formation of lower nuclearity iron carbonyl clusters.
Substitution: The carbonyl ligands in this compound can be substituted with other ligands such as phosphines or amines.
Common reagents used in these reactions include bases like triethylamine, acids like hydrochloric acid, and various ligands for substitution reactions . Major products formed from these reactions include diiron and tetrairon carbonyl complexes .
Scientific Research Applications
Catalytic Applications
1.1 Hydroboration Reactions
Triiron dodecacarbonyl has been effectively utilized as a catalyst in hydroboration reactions. Recent studies have demonstrated its ability to facilitate the selective reduction of esters to ethers, showcasing a wide substrate scope. This application is particularly valuable in organic synthesis where selective transformations are crucial .
1.2 Alkene Hydrosilylation
Another prominent application of this compound is in alkene hydrosilylation. Research indicates that this compound can enhance the efficiency of hydrosilylation reactions, which are essential for producing silanes and silicones used in various industrial applications .
Material Science
2.1 Chemical Vapor Deposition (CVD)
This compound is employed in chemical vapor deposition processes to create thin films of iron nanoparticles. These films exhibit desirable properties such as high surface area and catalytic activity, making them suitable for applications in electronics and sensors . The average size of the iron nanoparticles produced through this method is approximately 4.1 nm, indicating the potential for nanoscale applications.
2.2 Photocatalytic Applications
The compound has also been explored for photocatalytic applications, where it acts as a precursor for synthesizing other materials under light irradiation. This capability allows for the development of novel materials with tailored properties for energy conversion and storage technologies .
Polymer Synthesis
3.1 Synthesis of Polysiloxanes
This compound plays a role in the synthesis of polysiloxanes, where it can be involved in polymerization reactions that yield silicone-based materials with unique properties. The use of this compound in these processes can lead to higher yields and improved material characteristics .
Summary Table of Applications
Application Area | Specific Use Case | Key Benefits |
---|---|---|
Catalysis | Hydroboration | Selective reduction of esters to ethers |
Alkene hydrosilylation | Enhanced efficiency in producing silanes | |
Material Science | Chemical Vapor Deposition | Creation of iron nanoparticle films |
Photocatalytic applications | Development of materials for energy conversion | |
Polymer Synthesis | Synthesis of polysiloxanes | Higher yields and improved material properties |
Case Studies
Case Study 1: Hydroboration Efficiency
In a study published by ACS Publications, researchers demonstrated that this compound significantly improved the yield and selectivity in hydroboration reactions compared to traditional catalysts. The findings indicated that varying reaction conditions could further optimize performance, making it a valuable tool in synthetic organic chemistry .
Case Study 2: Thin Film Production via CVD
A research article highlighted the use of this compound in CVD processes to produce thin films of iron nanoparticles. The resulting films were characterized using various techniques, confirming their suitability for electronic applications due to their high conductivity and catalytic properties .
Mechanism of Action
The mechanism by which triiron dodecacarbonyl exerts its effects involves the coordination of its iron atoms with various ligands. The compound’s triangular structure allows for unique interactions with other molecules, facilitating reactions such as ligand substitution and bond cleavage . The molecular targets and pathways involved in these reactions are primarily related to the iron centers and their ability to coordinate with different ligands .
Comparison with Similar Compounds
Triiron dodecacarbonyl can be compared with other similar compounds, such as:
Triruthenium Dodecacarbonyl (Ru₃(CO)₁₂): Similar in structure but contains ruthenium instead of iron.
Triosmium Dodecacarbonyl (Os₃(CO)₁₂): Another analogous compound with osmium.
Iron Pentacarbonyl (Fe(CO)₅): A simpler iron carbonyl compound with five carbonyl ligands.
This compound is unique due to its triangular structure and the specific reactivity of its iron centers, which make it a versatile compound in various chemical reactions and applications .
Biological Activity
Triiron dodecacarbonyl (Fe₃(CO)₁₂) is an organoiron compound notable for its unique structure and reactivity, particularly in biological contexts. This article explores its biological activity, synthesis, reactivity, and potential applications, supported by data tables and case studies.
Structure and Properties
This compound consists of three iron atoms arranged in a triangular configuration, surrounded by twelve carbon monoxide (CO) ligands. The compound appears as a dark green solid that sublimates under vacuum and is soluble in nonpolar organic solvents . Its structure can be represented as follows:
The compound is known for its reactivity, especially as a source of zero-valent iron, which can participate in various chemical reactions, including those mimicking biological processes.
Synthesis
The synthesis of this compound typically involves the thermolysis of iron pentacarbonyl (Fe(CO)₅) or the reaction of Fe(CO)₅ with a base followed by acid treatment. The general reaction pathway is:
This synthesis route highlights the compound's accessibility for research and potential applications in catalysis and biomimetic chemistry.
Hydrogenase Mimics
One of the most promising areas of research involving this compound is its role as a bioinspired model for hydrogenases. These enzymes are crucial for hydrogen production and utilization in biological systems. Studies have shown that this compound can effectively catalyze proton reduction, mimicking the function of natural hydrogenases .
Case Study: Catalytic Activity
A study demonstrated that this compound complexes modified with bisphosphine ligands exhibited significant catalytic activity for proton reduction at low overpotential. The catalytic cycle involved the formation of stable iron-sulfur clusters that facilitated electron transfer processes analogous to those occurring in natural hydrogenases.
Interaction with Biological Molecules
This compound has also been investigated for its interactions with various biological molecules. For instance, research indicates that it can form π-complexes with fatty acids such as conjugated linoleic acid (CLA), enhancing our understanding of its potential roles in biochemical pathways .
Reactivity and Safety Considerations
While this compound shows great promise in biological applications, it also poses safety risks due to its pyrophoric nature and toxicity associated with carbon monoxide release. Proper handling protocols are essential when working with this compound in laboratory settings.
Summary of Key Findings
Aspect | Details |
---|---|
Chemical Formula | Fe₃(CO)₁₂ |
Structure | Triangular arrangement of three iron atoms with twelve CO ligands |
Synthesis Methods | Thermolysis of Fe(CO)₅; reaction with bases and acids |
Biological Role | Mimics hydrogenases; catalyzes proton reduction |
Safety Risks | Pyrophoric; toxic carbon monoxide release |
Q & A
Q. Basic: What are the established synthesis methods for triiron dodecacarbonyl, and what parameters critically influence yield?
This compound is typically synthesized via thermal decomposition of diiron nonacarbonyl (Fe₂(CO)₉) in toluene at 70°C . An alternative method involves reacting Fe(CO)₅ with a base (e.g., aqueous hydroxide) followed by acid oxidation of the intermediate hydride (HFe(CO)₄⁻) . Key parameters include reaction temperature (controlled to avoid decomposition), solvent purity (nonpolar solvents like toluene), and inert atmosphere (to prevent oxidation). Excess CO pressure may improve yield by stabilizing intermediates.
Q. Basic: What spectroscopic and analytical techniques are essential for characterizing Fe₃(CO)₁₂ and validating its purity?
- Infrared (IR) Spectroscopy : Identifies terminal (1950–2050 cm⁻¹) and bridging (1750–1850 cm⁻¹) CO ligands, confirming the C₂v symmetry of Fe₃(CO)₁₂ .
- Mößbauer Spectroscopy : Resolves inequivalent iron sites via quadrupole splitting (e.g., 1.13 and 0.13 mm/s coupling constants), critical for structural validation .
- X-ray Diffraction (XRD) : Resolves ligand disorder in crystallographic studies, though challenges arise due to CO ligand mobility .
- Elemental Analysis : Ensures stoichiometric ratios of Fe, C, and O.
Q. Advanced: How do structural discrepancies between Fe₃(CO)₁₂ and Ru₃(CO)₁₂/Os₃(CO)₁₂ impact their reactivity in catalytic applications?
Fe₃(CO)₁₂ adopts a C₂v-symmetric structure with two bridging CO ligands, whereas Ru/Os analogs (D₃h symmetry) feature only terminal CO ligands. This structural difference lowers the activation energy for ligand substitution in Fe₃(CO)₁₂, enhancing its reactivity in catalytic reductions (e.g., nitro-to-amine conversions) under milder conditions . Comparative studies using kinetic assays (e.g., turnover frequency measurements) and computational modeling (DFT) reveal Fe₃(CO)₁₂’s superior electron-transfer capacity due to labile bridging CO ligands .
Q. Advanced: What mechanistic pathways explain Fe₃(CO)₁₂’s role in nanoparticle synthesis, and how do decomposition byproducts affect outcomes?
Fe₃(CO)₁₂ decomposes under thermal or photolytic conditions to form iron nanoparticles. The process involves CO dissociation, forming Fe clusters that aggregate into nanoparticles. Byproducts like CO gas and pyrophoric iron residues require careful handling (e.g., Schlenk-line techniques) . In advanced oxidation processes (AOPs), Fe₃(CO)₁₂-derived nanoparticles exhibit Fenton-like activity, with catalytic efficiency linked to surface defects and Fe²⁺/Fe³⁺ ratios, validated via XPS and TEM .
Q. Basic: What safety protocols are critical for handling Fe₃(CO)₁₂ in laboratory settings?
- Storage : Under inert gas (N₂/Ar) at 0–6°C to prevent air-sensitive decomposition .
- Decomposition Risks : Solid residues are pyrophoric; quenching with ethanol or dilute acid is recommended .
- CO Hazard Mitigation : Use fume hoods and CO detectors due to carbonyl toxicity .
Q. Advanced: How can contradictions in reported catalytic efficiencies of Fe₃(CO)₁₂ be resolved through experimental design?
Discrepancies often arise from solvent polarity (e.g., toluene vs. THF), which affects cluster solubility and ligand substitution rates. Controlled studies should:
- Standardize reaction conditions (solvent, temperature, catalyst loading).
- Use kinetic profiling (e.g., in situ IR to monitor CO ligand loss).
- Compare turnover numbers (TON) across studies while accounting for byproduct inhibition (e.g., CO buildup) .
Q. Basic: What are the primary applications of Fe₃(CO)₁₂ in organic synthesis?
Fe₃(CO)₁₂ serves as a precursor for:
- Reduction Catalysts : Efficient in nitro-to-amine conversions under mild conditions, outperforming Pd/C in substrates sensitive to hydrogenation .
- Cluster Synthesis : Forms higher-nuclearity clusters (e.g., Fe₅(CO)₁₅C) via disproportionation of CO .
Q. Advanced: What computational methods are employed to model Fe₃(CO)₁₂’s electronic structure and reaction pathways?
Density Functional Theory (DFT) simulations elucidate:
- Ligand Dynamics : Energy barriers for CO bridging-terminal isomerization.
- Reactive Intermediates : Hydride (HFe₃(CO)₁₁⁻) formation during catalytic cycles .
Validation via spectroscopic data (e.g., Mößbauer parameters) ensures model accuracy.
Q. Basic: How should researchers address inconsistencies in Fe₃(CO)₁₂ characterization data across literature sources?
- Reproducibility Checks : Replicate synthesis and characterization under cited conditions.
- Data Triangulation : Cross-reference XRD, IR, and Mößbauer data to confirm structural assignments .
- Metadata Reporting : Document solvent, temperature, and instrument calibration details to identify confounding variables .
Q. Advanced: What strategies optimize Fe₃(CO)₁₂’s stability in catalytic systems prone to cluster fragmentation?
Properties
IUPAC Name |
carbon monoxide;iron | |
---|---|---|
Source | PubChem | |
URL | https://pubchem.ncbi.nlm.nih.gov | |
Description | Data deposited in or computed by PubChem | |
InChI |
InChI=1S/12CO.3Fe/c12*1-2;;; | |
Source | PubChem | |
URL | https://pubchem.ncbi.nlm.nih.gov | |
Description | Data deposited in or computed by PubChem | |
InChI Key |
HLYRMDDXFDINCB-UHFFFAOYSA-N | |
Source | PubChem | |
URL | https://pubchem.ncbi.nlm.nih.gov | |
Description | Data deposited in or computed by PubChem | |
Canonical SMILES |
[C-]#[O+].[C-]#[O+].[C-]#[O+].[C-]#[O+].[C-]#[O+].[C-]#[O+].[C-]#[O+].[C-]#[O+].[C-]#[O+].[C-]#[O+].[C-]#[O+].[C-]#[O+].[Fe].[Fe].[Fe] | |
Source | PubChem | |
URL | https://pubchem.ncbi.nlm.nih.gov | |
Description | Data deposited in or computed by PubChem | |
Molecular Formula |
C12Fe3O12 | |
Source | PubChem | |
URL | https://pubchem.ncbi.nlm.nih.gov | |
Description | Data deposited in or computed by PubChem | |
Molecular Weight |
503.65 g/mol | |
Source | PubChem | |
URL | https://pubchem.ncbi.nlm.nih.gov | |
Description | Data deposited in or computed by PubChem | |
CAS No. |
17685-52-8 | |
Record name | Dodecacarbonyl iron | |
Source | ChemIDplus | |
URL | https://pubchem.ncbi.nlm.nih.gov/substance/?source=chemidplus&sourceid=0017685528 | |
Description | ChemIDplus is a free, web search system that provides access to the structure and nomenclature authority files used for the identification of chemical substances cited in National Library of Medicine (NLM) databases, including the TOXNET system. | |
Disclaimer and Information on In-Vitro Research Products
Please be aware that all articles and product information presented on BenchChem are intended solely for informational purposes. The products available for purchase on BenchChem are specifically designed for in-vitro studies, which are conducted outside of living organisms. In-vitro studies, derived from the Latin term "in glass," involve experiments performed in controlled laboratory settings using cells or tissues. It is important to note that these products are not categorized as medicines or drugs, and they have not received approval from the FDA for the prevention, treatment, or cure of any medical condition, ailment, or disease. We must emphasize that any form of bodily introduction of these products into humans or animals is strictly prohibited by law. It is essential to adhere to these guidelines to ensure compliance with legal and ethical standards in research and experimentation.