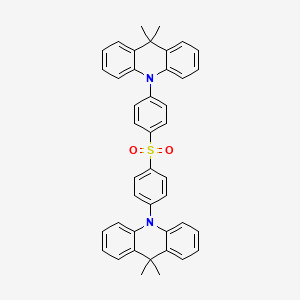
Dmac-dps
Overview
Description
Preparation Methods
Synthetic Routes and Reaction Conditions
The synthesis of DMAC-DPS involves a multi-step process. Initially, 10,10’-(sulfonylbis(4,1-phenylene))bis(2,7-diiodo-9,9-dimethyl-9,10-dihydroacridine) is prepared by iodization of this compound with N-iodosuccinimide. This intermediate is then coupled with 3,6-di-tert-butyl-9H-carbazole or 3,3’‘,6,6’‘-tetra-tert-butyl-9’H-9,3’:6’,9’'-tercarbazole through copper-catalyzed C-N cross-coupling reactions .
Industrial Production Methods
Industrial production of this compound typically involves large-scale synthesis using similar reaction conditions as in the laboratory. The process is optimized for higher yields and purity, ensuring the compound’s suitability for commercial applications in OLEDs .
Chemical Reactions Analysis
Synthetic Routes and Functionalization
DMAC-DPS is synthesized via a Cu-catalyzed C-N cross-coupling reaction between 5,5-dimethyl-5,10-dihydropyrido[2,3-b] naphthyridine (DMDHPN) and diphenylsulfone (DPS) derivatives. Key steps include:
-
Reactants : DMAC donor and DPS acceptor moieties.
-
Conditions : Conducted in tetrahydrofuran (THF) with Cu catalysts.
-
Outcome : High thermal stability (>450°C decomposition temperature) and glass transition temperatures (~272°C), enabling solution-processed OLED fabrication .
Reaction Component | Details |
---|---|
Donor Unit | DMAC (electron-rich) |
Acceptor Unit | DPS (electron-deficient) |
Solvent | THF or chlorobenzene |
Catalyst | Cu-based |
Yield | Confirmed via NMR and MALDI-TOF MS |
Photodegradation Mechanisms
This compound undergoes UV-induced degradation primarily at the triplet (T1) state, as demonstrated by:
-
Triplet State Susceptibility : Bond cleavage occurs at T1 due to low energy barriers (ΔEST ≈ 0.1–0.3 eV) .
-
Quenching Effects :
-
Film-State Instability : Delayed emission persists in films, leading to faster degradation compared to solutions .
Key Data :
-
Degradation Rate : Emission intensity drops by >50% after 5 hours of UV exposure in degassed solutions .
-
Activation Energy : Correlates linearly with bond dissociation energy (BDE) − T1 energy (R² = 0.98) .
Derivatization via Substitution
Chemical modifications to this compound alter its optoelectronic properties:
"CH/N" Substitution
-
Site : Diphenylsulfone (DPS) acceptor.
-
Effect : Reduces singlet-triplet gap (ΔEST) to 0.07 eV, enhancing reverse intersystem crossing (RISC) .
"H/CN" Substitution
-
Site : Ortho/meta positions of DPS.
-
Effect :
Substitution Type | ΔEST (eV) | λem (nm) | PLQY (%) |
---|---|---|---|
Parent this compound | 0.10 | 492 | 68 |
CH/N | 0.07 | 464 | 48 |
H/CN (ortho) | 0.05 | 449 | 55 |
Electrochemical Behavior
This compound exhibits quasi-reversible redox processes :
CV Data :
Process | Potential (V vs. SCE) | Assignment |
---|---|---|
Oxidation 1 | +1.11 | DMAC unit |
Oxidation 2 | +1.30 | Carbazole dendron |
Reduction | −1.64 | DPS unit |
Host-Dopant Interactions
This compound shows enhanced photoluminescence quantum yields (PLQYs) in specific hosts:
Scientific Research Applications
Key Applications
-
Organic Light-Emitting Diodes (OLEDs)
- DMAC-DPS is primarily used in OLEDs due to its ability to enhance light emission efficiency. It acts as a host material that supports guest dyes, such as C545T, facilitating energy transfer processes that lead to improved device performance.
- Research indicates that OLEDs utilizing this compound can achieve high external quantum efficiencies and reduced roll-off effects at high currents, making them suitable for display technologies .
-
Thermally Activated Delayed Fluorescence (TADF)
- The TADF mechanism enabled by this compound allows for the harvesting of triplet excitons, which are typically lost in traditional fluorescent materials. This characteristic significantly boosts the overall efficiency of OLED devices.
- Studies have shown that this compound can achieve delayed lifetimes on the order of microseconds, enhancing the energy transfer efficiency from the host to the dopant materials .
- Doping-Free Phosphorescent OLEDs
Case Study 1: High-Efficiency Green OLEDs
A study conducted on green OLEDs incorporating this compound revealed that it exhibited two distinct lifetimes—prompt and delayed—due to efficient reverse intersystem crossing (RISC). The research highlighted how varying concentrations of guest materials affected the delayed lifetime, ultimately optimizing the emission characteristics of the device .
Case Study 2: First-Principles Calculations
An investigation using first-principles calculations focused on this compound and related compounds demonstrated their potential for superior performance in OLED applications. The study utilized time-dependent density functional theory to analyze optical spectra and established a strong correlation between theoretical predictions and experimental results .
Comparative Data Table
Property | This compound Characteristics | Application Impact |
---|---|---|
Type | TADF material | Enhances light emission efficiency |
Emission Color | Blue | Suitable for blue OLED applications |
Delayed Lifetime | Up to 3 μs | Improves energy transfer efficiency |
Quantum Efficiency | High | Reduces roll-off at high currents |
Device Architecture | Doping-free phosphorescent OLEDs | Simplifies design while maintaining performance |
Mechanism of Action
The mechanism of action of DMAC-DPS involves the reverse intersystem crossing (RISC) process, where non-radiative triplet excitons are converted into radiative singlet excitons. This process is facilitated by the well-separated highest occupied molecular orbital (HOMO) and lowest unoccupied molecular orbital (LUMO) distributions on the DMAC and DPS units, respectively .
Comparison with Similar Compounds
Similar Compounds
CzDMAC-DPS: A derivative with carbazole dendrons, exhibiting a red-shifted emission peak.
DCzthis compound: Another derivative with different carbazole substitutions, showing unique photophysical properties.
ACXA-DPS: A compound with a smaller singlet-triplet energy gap, suitable for deep blue emission.
Uniqueness
This compound stands out due to its high photoluminescence quantum yield and efficient TADF properties, making it a preferred choice for blue OLED emitters .
Biological Activity
DMAC-DPS, a compound derived from diphenylsulfone and dimethylaminocinnamaldehyde, has garnered attention for its biological activity, particularly in the context of organic light-emitting diodes (OLEDs) and photophysical properties. This article provides a detailed overview of the biological activity of this compound, supported by data tables and relevant research findings.
Overview of this compound
This compound is characterized as a thermally activated delayed fluorescence (TADF) material, which is crucial for enhancing the efficiency of OLEDs. Its unique molecular structure allows it to exhibit significant photophysical properties, including high photoluminescence quantum yield (PLQY) and efficient energy transfer mechanisms.
Photophysical Properties
The photophysical properties of this compound have been extensively studied through various theoretical and experimental approaches. The following table summarizes key photophysical parameters:
Property | Value |
---|---|
Emission Wavelength | ~460 nm |
PLQY | ~70% |
Delayed Fluorescence Lifetime () | 3.3 µs in toluene |
Quantum Yield of Delayed Fluorescence () | 93% in toluene |
Dexter Energy Transfer Rate Constant () |
These properties indicate that this compound is an efficient emitter suitable for various applications in organic electronics.
Mechanisms of Biological Activity
1. Iron Homeostasis:
Research indicates that proteins similar to this compound play a role in iron homeostasis within bacterial systems. For instance, DNA binding proteins under starvation (Dps) are known to store iron and protect DNA from oxidative damage, which is essential for cellular survival during stress conditions .
2. Photodegradation Resistance:
Studies have shown that this compound exhibits notable resistance to photodegradation when incorporated into polymer films. For example, films containing 40 wt% this compound demonstrated significant stability against UV-induced degradation over time . This property is critical for maintaining the longevity and performance of OLED devices.
3. Reactive Oxygen Species (ROS) Scavenging:
The ability of this compound to mitigate oxidative stress through ROS scavenging has been highlighted in various studies. This activity is vital for protecting cellular components from damage caused by free radicals, thereby contributing to overall cellular health.
Case Studies
Case Study 1: OLED Performance Enhancement
In a study examining the effects of this compound on OLED performance, researchers found that incorporating this compound as a sensitizer significantly improved the external quantum efficiency (EQE) of devices. The maximum EQE observed was 25.2%, demonstrating the compound's effectiveness in enhancing light emission without compromising color purity .
Case Study 2: Photostability Assessment
A comparative analysis of different TADF materials, including this compound, revealed that films doped with this compound maintained their luminescent properties better than other materials under prolonged UV exposure. This highlights its potential application in environments where light stability is crucial .
Q & A
Q. How should experimental designs using DMAC-DPS align with FAIR data principles to ensure reproducibility?
Level: Basic
Methodological Answer:
When designing experiments with this compound, researchers should structure workflows to adhere to FAIR (Findable, Accessible, Interoperable, Reusable) principles. For example:
- Findability: Use persistent identifiers (DOIs) for datasets generated in DPS and embed metadata templates in the Data Management Plan (DMP) .
- Interoperability: Standardize data formats (e.g., CSV, XML) compatible with community repositories and DPS’s statistical modules .
- Reusability: Document experimental parameters (e.g., sample sizes, control variables) in the DMP to enable replication .
DMPs should reference domain-specific repositories (e.g., Zenodo for entomology) to ensure long-term accessibility .
Q. What advanced methodologies resolve data contradictions in this compound statistical outputs?
Level: Advanced
Methodological Answer:
Contradictions may arise from heterogeneous datasets or algorithmic biases. To address this:
- Sensitivity Analysis: Use DPS’s built-in Monte Carlo simulations to test robustness of statistical models under varying assumptions .
- Meta-Analysis: Cross-validate results with independent datasets archived in repositories cited in the DMP .
- Error Tracing: Document raw data preprocessing steps (e.g., outlier removal criteria) in the DMP to isolate discrepancies .
For peer review, provide machine-actionable DMPs that link analysis scripts to raw data .
Q. How can this compound workflows be integrated into institutional DMP templates?
Level: Basic
Methodological Answer:
- Template Selection: Use tools like DMPonline or Data Stewardship Wizard, which provide customizable DMP frameworks aligned with funding agency requirements (e.g., DOE, MRC) .
- Data Flow Mapping: In the DMP, specify how raw data from DPS experiments will be transferred to secure storage (e.g., institutional repositories with ISO 27001 compliance) .
- Metadata Standards: Adopt domain-specific schemas (e.g., Darwin Core for biodiversity data) to ensure compatibility with DPS outputs .
Q. How to validate this compound findings when data sharing is restricted due to ethical constraints?
Level: Advanced
Methodological Answer:
- Synthetic Data: Generate anonymized datasets using DPS’s data masking tools to replicate original distributions without exposing sensitive information .
- Third-Party Audits: Partner with independent labs to rerun analyses using DPS scripts under controlled access, as outlined in the DMP’s confidentiality protocols .
- Partial Sharing: Release aggregated results or metadata in public repositories, with access to raw data contingent on ethical review board approval .
Q. What criteria determine data type prioritization in DMPs for this compound-driven studies?
Level: Basic
Methodological Answer:
- Scientific Value: Prioritize data essential for validating hypotheses (e.g., raw measurements over derived metrics) .
- Cost-Benefit Analysis: Justify exclusion of low-impact data (e.g., redundant replicates) in the DMP based on storage costs and reuse potential .
- Community Standards: Align with field-specific guidelines (e.g., MIAME for microarray data) to ensure interoperability .
Q. How to address ethical challenges in sharing sensitive data from this compound studies?
Level: Advanced
Methodological Answer:
- GDPR Compliance: Implement DPS’s pseudonymization features to strip personally identifiable information (PII) before archiving .
- Controlled Access: Use repositories with embargo options (e.g., ICPSR) and detail access protocols in the DMP .
- Ethical Review: Collaborate with institutional review boards (IRBs) to define data-sharing boundaries, documented in the DMP’s ethics section .
Q. What steps enable machine-actionable DMPs for this compound projects?
Level: Advanced
Methodological Answer:
- Semantic Tagging: Use DMP Common Standard Ontology to structure metadata, enabling automated validation by funders .
- API Integration: Link DMPs to DPS via REST APIs for real-time updates on data collection and analysis milestones .
- Format Standardization: Export DMPs in JSON-LD or XML to ensure compatibility with grant management systems .
Q. How does this compound enhance reproducibility in longitudinal studies?
Level: Basic
Methodological Answer:
- Version Control: Use DPS’s built-in Git integration to track changes in analytical workflows .
- Dynamic DMPs: Update DMPs quarterly to reflect methodological adjustments (e.g., sampling intervals), ensuring alignment with peer-review expectations .
- Data Provenance: Embed timestamps and contributor roles in metadata to clarify data lineage .
Q. What strategies reconcile conflicting datasets in this compound meta-analyses?
Level: Advanced
Methodological Answer:
- Hierarchical Modeling: Apply DPS’s mixed-effects models to account for variability across studies .
- Bias Adjustment: Use inverse-variance weighting in DPS to normalize heterogeneous data .
- Transparency: Publish preprocessing code and conflict resolution criteria in the DMP’s appendix .
Q. Which DMP frameworks are recommended for this compound studies in interdisciplinary contexts?
Level: Basic
Methodological Answer:
- Funding-Aligned Templates: Use the DOE/SC DMP template for energy research or MRC’s template for biomedical studies .
- Customization Tools: Leverage DMPTool to merge discipline-specific requirements (e.g., ecological + computational data) .
- Cross-Referencing: Cite FAIRsharing.org resources in the DMP to ensure compliance with multiple domain standards .
Properties
IUPAC Name |
10-[4-[4-(9,9-dimethylacridin-10-yl)phenyl]sulfonylphenyl]-9,9-dimethylacridine | |
---|---|---|
Details | Computed by Lexichem TK 2.7.0 (PubChem release 2021.05.07) | |
Source | PubChem | |
URL | https://pubchem.ncbi.nlm.nih.gov | |
Description | Data deposited in or computed by PubChem | |
InChI |
InChI=1S/C42H36N2O2S/c1-41(2)33-13-5-9-17-37(33)43(38-18-10-6-14-34(38)41)29-21-25-31(26-22-29)47(45,46)32-27-23-30(24-28-32)44-39-19-11-7-15-35(39)42(3,4)36-16-8-12-20-40(36)44/h5-28H,1-4H3 | |
Details | Computed by InChI 1.0.6 (PubChem release 2021.05.07) | |
Source | PubChem | |
URL | https://pubchem.ncbi.nlm.nih.gov | |
Description | Data deposited in or computed by PubChem | |
InChI Key |
CYPVTICNYNXTQP-UHFFFAOYSA-N | |
Details | Computed by InChI 1.0.6 (PubChem release 2021.05.07) | |
Source | PubChem | |
URL | https://pubchem.ncbi.nlm.nih.gov | |
Description | Data deposited in or computed by PubChem | |
Canonical SMILES |
CC1(C2=CC=CC=C2N(C3=CC=CC=C31)C4=CC=C(C=C4)S(=O)(=O)C5=CC=C(C=C5)N6C7=CC=CC=C7C(C8=CC=CC=C86)(C)C)C | |
Details | Computed by OEChem 2.3.0 (PubChem release 2021.05.07) | |
Source | PubChem | |
URL | https://pubchem.ncbi.nlm.nih.gov | |
Description | Data deposited in or computed by PubChem | |
Molecular Formula |
C42H36N2O2S | |
Details | Computed by PubChem 2.1 (PubChem release 2021.05.07) | |
Source | PubChem | |
URL | https://pubchem.ncbi.nlm.nih.gov | |
Description | Data deposited in or computed by PubChem | |
Molecular Weight |
632.8 g/mol | |
Details | Computed by PubChem 2.1 (PubChem release 2021.05.07) | |
Source | PubChem | |
URL | https://pubchem.ncbi.nlm.nih.gov | |
Description | Data deposited in or computed by PubChem | |
Disclaimer and Information on In-Vitro Research Products
Please be aware that all articles and product information presented on BenchChem are intended solely for informational purposes. The products available for purchase on BenchChem are specifically designed for in-vitro studies, which are conducted outside of living organisms. In-vitro studies, derived from the Latin term "in glass," involve experiments performed in controlled laboratory settings using cells or tissues. It is important to note that these products are not categorized as medicines or drugs, and they have not received approval from the FDA for the prevention, treatment, or cure of any medical condition, ailment, or disease. We must emphasize that any form of bodily introduction of these products into humans or animals is strictly prohibited by law. It is essential to adhere to these guidelines to ensure compliance with legal and ethical standards in research and experimentation.