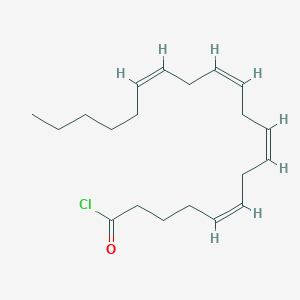
Arachidonoyl chloride
- Click on QUICK INQUIRY to receive a quote from our team of experts.
- With the quality product at a COMPETITIVE price, you can focus more on your research.
Description
Arachidonoyl chloride is a derivative of arachidonic acid. It has been used as an intermediate in the synthesis of arachidonic acid derivatives.
Mechanism of Action
Target of Action
Arachidonoyl chloride is a derivative of arachidonic acid . It is primarily involved in the endocannabinoid system, where it interacts with various receptors, including the cannabinoid receptors .
Mode of Action
This compound’s interaction with its targets results in various changes. For instance, it plays a role in the synthesis of anandamide (AEA) and 2-arachidonoylglycerol (2-AG), two major endogenous agonists of the cannabinoid receptors . These molecules are engaged in different forms of synaptic plasticity and take part in different behavioral functions .
Biochemical Pathways
This compound is involved in the biosynthesis of endocannabinoids. The metabolic pathways of these endocannabinoids are completely different. For instance, 2-AG is mostly produced from inositol phospholipids via diacylglycerol by phospholipase C and diacylglycerol lipase and then degraded by monoacylglycerol lipase . On the other hand, anandamide is concomitantly produced with larger amounts of other N-acylethanolamines via N-acyl-phosphatidylethanolamines (NAPEs) .
Pharmacokinetics
It is known that anandamide and 2-ag, which are synthesized from this compound, are both synthesized on demand and have a short-lived transmission .
Result of Action
The molecular and cellular effects of this compound’s action are primarily mediated through its role in the synthesis of endocannabinoids. These endocannabinoids have various effects, including the resolution of acute pain, analgesic benefit to neuropathic, gastrointestinal, inflammatory, and chemotherapy-induced pain .
Biochemical Analysis
Biochemical Properties
Arachidonoyl chloride interacts with various enzymes, proteins, and other biomolecules. It is used in biological studies for receptor binding and enzyme activities . It is a chemical compound that belongs to the group of amides . The carbonyl chloride group readily reacts with various nucleophiles, enabling efficient and controlled conjugation of bioactive molecules .
Cellular Effects
This compound plays a significant role in cellular processes. It has been used as an intermediate in the synthesis of arachidonic acid derivatives . It influences cell function, including any impact on cell signaling pathways, gene expression, and cellular metabolism .
Molecular Mechanism
This compound exerts its effects at the molecular level through various mechanisms. For instance, it has been shown to be involved in the activation of the human 5-lipoxygenase molecular mechanism of arachidonic acid . This involves binding interactions with biomolecules, enzyme inhibition or activation, and changes in gene expression .
Metabolic Pathways
This compound is involved in various metabolic pathways. For instance, it is known that arachidonic acid, from which this compound is derived, is converted into eicosanoids that promote inflammation, blood clotting, and vasoconstriction, and epoxyeicosatrienoic (EET), which have vasodilatory and anti-inflammatory effects .
Properties
IUPAC Name |
(5Z,8Z,11Z,14Z)-icosa-5,8,11,14-tetraenoyl chloride |
Source
|
---|---|---|
Details | Computed by LexiChem 2.6.6 (PubChem release 2019.06.18) | |
Source | PubChem | |
URL | https://pubchem.ncbi.nlm.nih.gov | |
Description | Data deposited in or computed by PubChem | |
InChI |
InChI=1S/C20H31ClO/c1-2-3-4-5-6-7-8-9-10-11-12-13-14-15-16-17-18-19-20(21)22/h6-7,9-10,12-13,15-16H,2-5,8,11,14,17-19H2,1H3/b7-6-,10-9-,13-12-,16-15- |
Source
|
Details | Computed by InChI 1.0.5 (PubChem release 2019.06.18) | |
Source | PubChem | |
URL | https://pubchem.ncbi.nlm.nih.gov | |
Description | Data deposited in or computed by PubChem | |
InChI Key |
NTBLHFFUSJRJQO-DOFZRALJSA-N |
Source
|
Details | Computed by InChI 1.0.5 (PubChem release 2019.06.18) | |
Source | PubChem | |
URL | https://pubchem.ncbi.nlm.nih.gov | |
Description | Data deposited in or computed by PubChem | |
Canonical SMILES |
CCCCCC=CCC=CCC=CCC=CCCCC(=O)Cl |
Source
|
Details | Computed by OEChem 2.1.5 (PubChem release 2019.06.18) | |
Source | PubChem | |
URL | https://pubchem.ncbi.nlm.nih.gov | |
Description | Data deposited in or computed by PubChem | |
Isomeric SMILES |
CCCCC/C=C\C/C=C\C/C=C\C/C=C\CCCC(=O)Cl |
Source
|
Details | Computed by OEChem 2.1.5 (PubChem release 2019.06.18) | |
Source | PubChem | |
URL | https://pubchem.ncbi.nlm.nih.gov | |
Description | Data deposited in or computed by PubChem | |
Molecular Formula |
C20H31ClO |
Source
|
Details | Computed by PubChem 2.1 (PubChem release 2019.06.18) | |
Source | PubChem | |
URL | https://pubchem.ncbi.nlm.nih.gov | |
Description | Data deposited in or computed by PubChem | |
Molecular Weight |
322.9 g/mol |
Source
|
Details | Computed by PubChem 2.1 (PubChem release 2021.05.07) | |
Source | PubChem | |
URL | https://pubchem.ncbi.nlm.nih.gov | |
Description | Data deposited in or computed by PubChem | |
Disclaimer and Information on In-Vitro Research Products
Please be aware that all articles and product information presented on BenchChem are intended solely for informational purposes. The products available for purchase on BenchChem are specifically designed for in-vitro studies, which are conducted outside of living organisms. In-vitro studies, derived from the Latin term "in glass," involve experiments performed in controlled laboratory settings using cells or tissues. It is important to note that these products are not categorized as medicines or drugs, and they have not received approval from the FDA for the prevention, treatment, or cure of any medical condition, ailment, or disease. We must emphasize that any form of bodily introduction of these products into humans or animals is strictly prohibited by law. It is essential to adhere to these guidelines to ensure compliance with legal and ethical standards in research and experimentation.