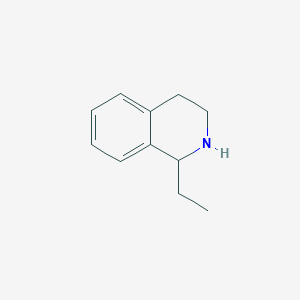
1-Ethyl-1,2,3,4-tetrahydroisoquinoline
Overview
Description
1-Ethyl-1,2,3,4-tetrahydroisoquinoline is a chemical compound belonging to the class of tetrahydroisoquinolines These compounds are characterized by their structural motif, which includes a partially saturated isoquinoline ring
Mechanism of Action
Target of Action
1-Ethyl-1,2,3,4-tetrahydroisoquinoline (1-Ethyl-THIQ) is a derivative of tetrahydroisoquinolines (THIQs), which are known to exert diverse biological activities against various infective pathogens and neurodegenerative disorders .
Mode of Action
Thiqs are known to interact with their targets in a variety of ways, including binding to active sites, allosteric modulation, and influencing signal transduction pathways . The specific interactions and resulting changes would depend on the particular target and the structural characteristics of the 1-Ethyl-THIQ molecule.
Biochemical Pathways
1-Ethyl-THIQ likely affects multiple biochemical pathways due to its structural similarity to THIQs. THIQs are known to influence various biochemical pathways, including those involved in neurotransmission, inflammation, and cellular metabolism . The downstream effects of these interactions can include changes in cellular function, modulation of immune responses, and alterations in neuronal activity .
Pharmacokinetics
Factors such as solubility, stability, and molecular size can influence how a compound is absorbed and distributed within the body, how it is metabolized by enzymes, and how it is ultimately excreted .
Result of Action
The molecular and cellular effects of 1-Ethyl-THIQ’s action would depend on its specific targets and mode of action. Given the diverse biological activities of THIQs, the effects could potentially include modulation of cellular signaling, alteration of enzyme activity, and changes in cellular function
Action Environment
Environmental factors can significantly influence the action, efficacy, and stability of a compound. Factors such as pH, temperature, and the presence of other molecules can affect a compound’s stability and its interactions with its targets . Additionally, the physiological environment within the body, including factors such as the presence of binding proteins, the characteristics of the cellular membrane, and the activity of metabolic enzymes, can also influence the action and efficacy of a compound .
Biochemical Analysis
Biochemical Properties
1-Ethyl-1,2,3,4-tetrahydroisoquinoline is a secondary amine with weakly basic properties and forms salts with strong acids . It can interact with various enzymes, proteins, and other biomolecules, exerting its effects through these interactions .
Cellular Effects
This compound has been found to have significant effects on various types of cells and cellular processes . It influences cell function, including impacts on cell signaling pathways, gene expression, and cellular metabolism .
Molecular Mechanism
The molecular mechanism of action of this compound involves binding interactions with biomolecules, enzyme inhibition or activation, and changes in gene expression . It exerts its effects at the molecular level, contributing to its diverse biological activities .
Temporal Effects in Laboratory Settings
The effects of this compound change over time in laboratory settings . Information on the product’s stability, degradation, and any long-term effects on cellular function observed in in vitro or in vivo studies is crucial for understanding its biochemical profile .
Dosage Effects in Animal Models
The effects of this compound vary with different dosages in animal models . Studies have observed threshold effects, as well as toxic or adverse effects at high doses .
Metabolic Pathways
This compound is involved in various metabolic pathways . It interacts with enzymes or cofactors, and can affect metabolic flux or metabolite levels .
Transport and Distribution
This compound is transported and distributed within cells and tissues . It interacts with transporters or binding proteins, and can affect its localization or accumulation .
Subcellular Localization
The subcellular localization of this compound and any effects on its activity or function are crucial aspects of its biochemical profile . This could include any targeting signals or post-translational modifications that direct it to specific compartments or organelles .
Preparation Methods
1-Ethyl-1,2,3,4-tetrahydroisoquinoline can be synthesized through several methods. One common synthetic route involves the Pictet-Spengler reaction, where a phenylethylamine derivative reacts with an aldehyde under acidic conditions to form the tetrahydroisoquinoline core . Another method involves the catalytic hydrogenation of isoquinoline derivatives in the presence of ethylating agents . Industrial production methods often utilize these reactions on a larger scale, optimizing conditions such as temperature, pressure, and catalyst concentration to achieve higher yields and purity.
Chemical Reactions Analysis
1-Ethyl-1,2,3,4-tetrahydroisoquinoline undergoes various chemical reactions, including:
Oxidation: This compound can be oxidized to form isoquinoline derivatives. Common oxidizing agents include potassium permanganate and chromium trioxide.
Reduction: Reduction reactions can convert the compound into more saturated derivatives. Hydrogenation using palladium on carbon is a typical method.
Substitution: Electrophilic substitution reactions can introduce various functional groups into the aromatic ring. Reagents such as halogens and nitrating agents are commonly used.
Scientific Research Applications
1-Ethyl-1,2,3,4-tetrahydroisoquinoline has several applications in scientific research:
Chemistry: It serves as a building block for the synthesis of complex organic molecules and natural product analogs.
Biology: The compound is used in studies related to neurotransmitter analogs and receptor binding assays.
Comparison with Similar Compounds
1-Ethyl-1,2,3,4-tetrahydroisoquinoline can be compared with other tetrahydroisoquinoline derivatives, such as:
1-Methyl-1,2,3,4-tetrahydroisoquinoline: Known for its neuroprotective properties and potential use in treating Parkinson’s disease.
1,2,3,4-Tetrahydroisoquinoline: The parent compound, widely studied for its biological activities and synthetic applications.
Ethyl 1,2,3,4-tetrahydro-1-isoquinolinecarboxylate: Used in the synthesis of various bioactive molecules.
Each of these compounds has unique properties and applications, making this compound a valuable addition to the family of tetrahydroisoquinolines.
Properties
IUPAC Name |
1-ethyl-1,2,3,4-tetrahydroisoquinoline | |
---|---|---|
Details | Computed by LexiChem 2.6.6 (PubChem release 2019.06.18) | |
Source | PubChem | |
URL | https://pubchem.ncbi.nlm.nih.gov | |
Description | Data deposited in or computed by PubChem | |
InChI |
InChI=1S/C11H15N/c1-2-11-10-6-4-3-5-9(10)7-8-12-11/h3-6,11-12H,2,7-8H2,1H3 | |
Details | Computed by InChI 1.0.5 (PubChem release 2019.06.18) | |
Source | PubChem | |
URL | https://pubchem.ncbi.nlm.nih.gov | |
Description | Data deposited in or computed by PubChem | |
InChI Key |
UDWVZWUSBKDYPY-UHFFFAOYSA-N | |
Details | Computed by InChI 1.0.5 (PubChem release 2019.06.18) | |
Source | PubChem | |
URL | https://pubchem.ncbi.nlm.nih.gov | |
Description | Data deposited in or computed by PubChem | |
Canonical SMILES |
CCC1C2=CC=CC=C2CCN1 | |
Details | Computed by OEChem 2.1.5 (PubChem release 2019.06.18) | |
Source | PubChem | |
URL | https://pubchem.ncbi.nlm.nih.gov | |
Description | Data deposited in or computed by PubChem | |
Molecular Formula |
C11H15N | |
Details | Computed by PubChem 2.1 (PubChem release 2019.06.18) | |
Source | PubChem | |
URL | https://pubchem.ncbi.nlm.nih.gov | |
Description | Data deposited in or computed by PubChem | |
Molecular Weight |
161.24 g/mol | |
Details | Computed by PubChem 2.1 (PubChem release 2021.05.07) | |
Source | PubChem | |
URL | https://pubchem.ncbi.nlm.nih.gov | |
Description | Data deposited in or computed by PubChem | |
Synthesis routes and methods
Procedure details
Disclaimer and Information on In-Vitro Research Products
Please be aware that all articles and product information presented on BenchChem are intended solely for informational purposes. The products available for purchase on BenchChem are specifically designed for in-vitro studies, which are conducted outside of living organisms. In-vitro studies, derived from the Latin term "in glass," involve experiments performed in controlled laboratory settings using cells or tissues. It is important to note that these products are not categorized as medicines or drugs, and they have not received approval from the FDA for the prevention, treatment, or cure of any medical condition, ailment, or disease. We must emphasize that any form of bodily introduction of these products into humans or animals is strictly prohibited by law. It is essential to adhere to these guidelines to ensure compliance with legal and ethical standards in research and experimentation.