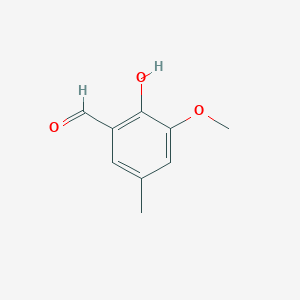
2-Hydroxy-3-methoxy-5-methylbenzaldehyde
- Click on QUICK INQUIRY to receive a quote from our team of experts.
- With the quality product at a COMPETITIVE price, you can focus more on your research.
Overview
Description
2-Hydroxy-3-methoxy-5-methylbenzaldehyde is a chemical compound with the molecular formula C9H10O3 . It has been used in various applications, including the synthesis of radiolabeling precursor desmethyl-PBR06 .
Synthesis Analysis
The synthesis of 2-Hydroxy-3-methoxy-5-methylbenzaldehyde has been achieved by heating p-cresol with hexamethylenetetramine . Another synthesis method involves the use of 2-hydroxy-5-methylisophthalaldehyde, a dialdehyde derivative .Molecular Structure Analysis
The molecular structure of 2-Hydroxy-3-methoxy-5-methylbenzaldehyde consists of a benzene ring substituted with hydroxy, methoxy, and methyl groups, as well as an aldehyde group . The InChI code for this compound is 1S/C9H10O3/c1-6-3-7(5-10)9(11)8(4-6)12-2/h3-5,11H,1-2H3 .Scientific Research Applications
1. Applications in Organic Synthesis and Chemical Reactions
2-Hydroxy-3-methoxy-5-methylbenzaldehyde has been utilized in various chemical synthesis processes. For instance, its anodic oxidation in acetonitrile-acetic acid results in an acetoxylated product, while the oxidation of its Schiff bases gives cyclohexenetriones (Ohmori, Matsumoto, & Masui, 1980). Additionally, it has been used as a starting material in the practical synthesis of complex compounds like ecteinascidin 743 and safracins, emphasizing its role in complex organic syntheses (Saito et al., 2000).
2. Role in Pheromone Synthesis and Chemical Ecology
The compound is also a significant component in the field of chemical ecology, specifically in the synthesis of pheromones. It has been used for the facile synthesis of 2-Hydroxy-6-methylbenzaldehyde, a compound functioning as an alarm and sex pheromone component in astigmatid mites (Noguchi, Mori, Kuwahara, & Sato, 1997).
3. Photoreactive Properties and Material Science Applications
Investigations into the photochemistry of o-tolualdehydes, including derivatives of 2-hydroxy-3-methoxy-5-methylbenzaldehyde, have revealed insights into their photoreactive properties. This understanding is crucial for potential applications in material science and photochemical transformations (Charlton & Koh, 1988).
4. Applications in Sensor Technology and Biological Research
A novel application of this compound is found in the development of fluorescent pH sensors. For example, a derivative of this compound, 3-[(3-benzyloxypyridin-2-ylimino)methyl]-2-hydroxy-5-methylbenzaldehyde, has been used as a highly selective fluorescent pH sensor, valuable for studying biological organelles (Saha et al., 2011).
Safety and Hazards
2-Hydroxy-3-methoxy-5-methylbenzaldehyde is considered hazardous according to the 2012 OSHA Hazard Communication Standard (29 CFR 1910.1200). It has been associated with acute oral toxicity, skin corrosion/irritation, serious eye damage/eye irritation, and specific target organ toxicity (single exposure), with the respiratory system being the target organ .
Mechanism of Action
Target of Action
It’s known that similar compounds often target proteins or enzymes involved in biochemical pathways .
Mode of Action
It’s known that similar compounds can react with other molecules to form new compounds . For instance, it can react with malononitrile to form 2-imino-6-methoxy-2H-1-benzopyran-3-carbonitrile .
Biochemical Pathways
Similar compounds are known to affect various biochemical pathways, leading to a range of downstream effects .
Pharmacokinetics
It’s known that similar compounds have high gastrointestinal absorption and are bbb permeant . These properties can significantly impact the compound’s bioavailability .
Result of Action
Similar compounds are known to have various effects at the molecular and cellular levels .
Action Environment
Environmental factors can influence the action, efficacy, and stability of 2-Hydroxy-3-methoxy-5-methylbenzaldehyde . For instance, it should be stored in a dry, cool, and well-ventilated place to maintain its stability .
properties
IUPAC Name |
2-hydroxy-3-methoxy-5-methylbenzaldehyde |
Source
|
---|---|---|
Details | Computed by LexiChem 2.6.6 (PubChem release 2019.06.18) | |
Source | PubChem | |
URL | https://pubchem.ncbi.nlm.nih.gov | |
Description | Data deposited in or computed by PubChem | |
InChI |
InChI=1S/C9H10O3/c1-6-3-7(5-10)9(11)8(4-6)12-2/h3-5,11H,1-2H3 |
Source
|
Details | Computed by InChI 1.0.5 (PubChem release 2019.06.18) | |
Source | PubChem | |
URL | https://pubchem.ncbi.nlm.nih.gov | |
Description | Data deposited in or computed by PubChem | |
InChI Key |
NDGVJTXAOLGFKK-UHFFFAOYSA-N |
Source
|
Details | Computed by InChI 1.0.5 (PubChem release 2019.06.18) | |
Source | PubChem | |
URL | https://pubchem.ncbi.nlm.nih.gov | |
Description | Data deposited in or computed by PubChem | |
Canonical SMILES |
CC1=CC(=C(C(=C1)OC)O)C=O |
Source
|
Details | Computed by OEChem 2.1.5 (PubChem release 2019.06.18) | |
Source | PubChem | |
URL | https://pubchem.ncbi.nlm.nih.gov | |
Description | Data deposited in or computed by PubChem | |
Molecular Formula |
C9H10O3 |
Source
|
Details | Computed by PubChem 2.1 (PubChem release 2019.06.18) | |
Source | PubChem | |
URL | https://pubchem.ncbi.nlm.nih.gov | |
Description | Data deposited in or computed by PubChem | |
Molecular Weight |
166.17 g/mol |
Source
|
Details | Computed by PubChem 2.1 (PubChem release 2021.05.07) | |
Source | PubChem | |
URL | https://pubchem.ncbi.nlm.nih.gov | |
Description | Data deposited in or computed by PubChem | |
Product Name |
2-Hydroxy-3-methoxy-5-methylbenzaldehyde |
Q & A
Q1: What is the key structural feature that influences the electrochemical behavior of 2-hydroxy-3-methoxy-5-methylbenzaldehyde and its Schiff base derivatives?
A1: The presence of the hydroxyl group (-OH) in 2-hydroxy-3-methoxy-5-methylbenzaldehyde plays a crucial role in its electrochemical behavior. [] During anodic oxidation, this hydroxyl group facilitates the formation of either a phenoxonium ion or a quinone methide intermediate, depending on the specific reaction conditions. [] These intermediates then react further, leading to the observed pyridination at either the ring or the side-chain methyl group. []
Q2: How does the introduction of a Schiff base influence the anodic oxidation of 2-hydroxy-3-methoxy-5-methylbenzaldehyde?
A2: Introducing a Schiff base to 2-hydroxy-3-methoxy-5-methylbenzaldehyde significantly alters its anodic oxidation pathway. [, , ] Instead of simple acetoxylation at the side-chain methyl group, as observed with the free aldehyde, [] the Schiff base derivatives primarily undergo cyclization reactions to form cyclohexenetriones upon oxidation. [] This difference arises from the imino group in the Schiff base, which participates in the reaction mechanism by promoting the formation of a quinone methide intermediate. []
Q3: Can you elaborate on the regioselectivity observed during the anodic pyridination of 2-hydroxy-3-methoxy-5-methylbenzaldehyde Schiff bases?
A3: Research shows that the anodic pyridination of 2-hydroxy-3-methoxy-5-methylbenzaldehyde Schiff bases exhibits remarkable regioselectivity. [] The pyridine moiety predominantly attaches to the aromatic ring, specifically at the position meta to the hydroxyl group and ortho to the imino group. [] This selectivity stems from the electronic and steric effects exerted by both the hydroxyl and imino groups within the molecule, guiding the incoming pyridine to the observed position. []
Q4: What analytical techniques were employed in these studies to characterize the reaction products and investigate the reaction mechanisms?
A4: Researchers utilized a combination of electrochemical techniques and spectroscopic methods to analyze the reactions. Cyclic voltammetry provided insights into the redox behavior of the compounds. [] Controlled potential and constant current electrolysis were employed to drive the reactions and isolate the products. [] The structures of the resulting compounds were then elucidated using spectroscopic techniques, likely including nuclear magnetic resonance (NMR) spectroscopy and mass spectrometry. [, , ]
Disclaimer and Information on In-Vitro Research Products
Please be aware that all articles and product information presented on BenchChem are intended solely for informational purposes. The products available for purchase on BenchChem are specifically designed for in-vitro studies, which are conducted outside of living organisms. In-vitro studies, derived from the Latin term "in glass," involve experiments performed in controlled laboratory settings using cells or tissues. It is important to note that these products are not categorized as medicines or drugs, and they have not received approval from the FDA for the prevention, treatment, or cure of any medical condition, ailment, or disease. We must emphasize that any form of bodily introduction of these products into humans or animals is strictly prohibited by law. It is essential to adhere to these guidelines to ensure compliance with legal and ethical standards in research and experimentation.