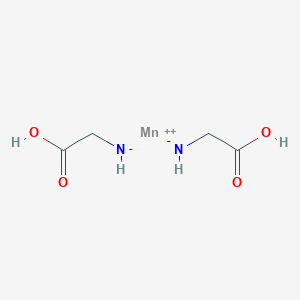
Manganese glycinate
Overview
Description
Manganese glycinate is a chelated form of manganese, where manganese is bound to the amino acid glycine. This compound is often used as a dietary supplement to provide the essential trace element manganese, which plays a crucial role in various physiological processes. Manganese is vital for the functioning of several enzymes, including manganese superoxide dismutase, which is an important antioxidant enzyme. This compound is known for its high bioavailability and stability, making it an effective source of manganese for nutritional and therapeutic purposes .
Mechanism of Action
- Primary Targets : Manganese glycinate dissociates in body fluids to form manganese cations (Mn²⁺) and gluconic acid. The pharmacological effects are primarily due to the normal role of manganese cations in the body .
Target of Action
Mode of Action
Preparation Methods
Synthetic Routes and Reaction Conditions: Manganese glycinate can be synthesized by reacting manganese salts, such as manganese sulfate or manganese chloride, with glycine in an aqueous solution. The reaction typically involves dissolving the manganese salt and glycine in water, adjusting the pH to around 6-7 using an alkaline pH buffering agent, and maintaining the temperature between 70-85°C. The reaction mixture is then allowed to react at constant temperature, followed by cooling, crystallization, and centrifugal separation to obtain this compound .
Industrial Production Methods: In industrial settings, the production of this compound involves similar steps but on a larger scale. The process may include additional steps such as spray drying to obtain a fine powder form of the compound. The use of calcium sources like calcium oxide or calcium hydroxide can help in precipitating sulfate radicals, thus improving the purity and yield of the final product .
Chemical Reactions Analysis
Types of Reactions: Manganese glycinate undergoes various chemical reactions, including:
Oxidation: Manganese in this compound can be oxidized to higher oxidation states.
Reduction: The compound can also undergo reduction reactions, particularly in the presence of reducing agents.
Substitution: this compound can participate in substitution reactions where the glycine ligand is replaced by other ligands.
Common Reagents and Conditions:
Oxidation: Common oxidizing agents include hydrogen peroxide and potassium permanganate.
Reduction: Reducing agents such as sodium borohydride and hydrogen gas can be used.
Substitution: Ligands like ethylenediaminetetraacetic acid (EDTA) can be used for substitution reactions.
Major Products Formed:
Oxidation: Higher oxidation state manganese compounds.
Reduction: Lower oxidation state manganese compounds.
Substitution: Complexes with different ligands.
Scientific Research Applications
Manganese glycinate has a wide range of applications in scientific research:
Chemistry: It is used as a precursor for the synthesis of other manganese complexes and as a catalyst in various chemical reactions.
Biology: this compound is studied for its role in enzymatic reactions and its potential to mitigate oxidative stress.
Medicine: It is used in dietary supplements to treat manganese deficiency and is being researched for its potential neuroprotective effects.
Industry: The compound is used in animal feed to ensure adequate manganese intake, which is essential for growth and development
Comparison with Similar Compounds
Manganese gluconate: Another chelated form of manganese, known for its high bioavailability and use in dietary supplements.
Manganese sulfate: A commonly used manganese supplement with good bioavailability but less stability compared to manganese glycinate.
Manganese chloride: Used in various industrial applications but less commonly in dietary supplements due to its lower bioavailability.
Uniqueness of this compound: this compound is unique due to its high bioavailability, stability, and ease of absorption in the body. Compared to other manganese supplements, it is less likely to cause gastrointestinal discomfort and is more effective in correcting manganese deficiency .
Biological Activity
Manganese glycinate, a chelated form of manganese, combines manganese with the amino acid glycine. This compound is gaining attention due to its potential biological activities and health benefits. This article explores the biological activity of this compound, focusing on its roles in metabolism, antioxidant defense, and potential therapeutic applications.
Overview of Manganese
Manganese is an essential trace mineral that plays a crucial role in various physiological processes. It acts as a cofactor for several enzymes involved in:
- Metabolism : Manganese is vital for carbohydrate and lipid metabolism, influencing glucose homeostasis and insulin secretion.
- Antioxidant Defense : It is a component of superoxide dismutase (SOD), an enzyme that protects cells from oxidative stress by catalyzing the dismutation of superoxide radicals into oxygen and hydrogen peroxide.
- Bone Health : Manganese contributes to bone formation and maintenance by participating in collagen synthesis and mineralization .
1. Metabolic Functions
This compound enhances the bioavailability of manganese compared to other forms, facilitating its absorption in the gastrointestinal tract. This increased bioavailability supports its role in metabolic pathways, particularly in glucose metabolism. Studies have indicated that manganese supplementation may improve insulin sensitivity and glucose tolerance, which is particularly beneficial for individuals with type 2 diabetes .
2. Antioxidant Properties
As a precursor to manganese superoxide dismutase (MnSOD), this compound plays a significant role in mitigating oxidative stress. Research has shown that adequate manganese levels can enhance SOD activity, thereby reducing cellular damage caused by free radicals. This antioxidant activity is crucial in managing inflammatory conditions and may contribute to pain relief in diseases such as osteoarthritis .
3. Bone Health
This compound has been linked to improved bone density and health. It aids in the formation of connective tissue and bone matrix, potentially reducing the risk of osteoporosis, particularly in postmenopausal women . A study indicated that supplementation with manganese could help maintain spinal bone density when combined with calcium and other minerals .
Case Study: Diabetes Management
A case-control study involving 1,614 adults with type 2 diabetes demonstrated a U-shaped relationship between plasma manganese levels and diabetes prevalence. Those with both low and high plasma manganese levels exhibited higher risks of diabetes compared to those with moderate levels .
Research Findings on Antioxidant Activity
A study involving patients with rheumatoid arthritis suggested that lower SOD activity correlates with disease severity. Supplementing with manganese may help restore SOD function, highlighting its potential as an adjunct therapy for inflammatory diseases .
Comparative Analysis of this compound
Property | This compound | Other Forms (e.g., Manganese Sulfate) |
---|---|---|
Bioavailability | High | Moderate to Low |
Absorption Rate | Efficient | Less efficient |
Gastrointestinal Tolerance | Well tolerated | May cause gastrointestinal distress |
Role in Metabolism | Supports glucose metabolism | Limited evidence |
Antioxidant Function | Strong | Variable |
Properties
IUPAC Name |
2-aminoacetate;manganese(2+) | |
---|---|---|
Source | PubChem | |
URL | https://pubchem.ncbi.nlm.nih.gov | |
Description | Data deposited in or computed by PubChem | |
InChI |
InChI=1S/2C2H5NO2.Mn/c2*3-1-2(4)5;/h2*1,3H2,(H,4,5);/q;;+2/p-2 | |
Source | PubChem | |
URL | https://pubchem.ncbi.nlm.nih.gov | |
Description | Data deposited in or computed by PubChem | |
InChI Key |
JNMKPXXKHWQWFB-UHFFFAOYSA-L | |
Source | PubChem | |
URL | https://pubchem.ncbi.nlm.nih.gov | |
Description | Data deposited in or computed by PubChem | |
Canonical SMILES |
C(C(=O)[O-])N.C(C(=O)[O-])N.[Mn+2] | |
Source | PubChem | |
URL | https://pubchem.ncbi.nlm.nih.gov | |
Description | Data deposited in or computed by PubChem | |
Molecular Formula |
C4H8MnN2O4 | |
Source | PubChem | |
URL | https://pubchem.ncbi.nlm.nih.gov | |
Description | Data deposited in or computed by PubChem | |
DSSTOX Substance ID |
DTXSID10219181 | |
Record name | Manganese(II) bis(glycinate) | |
Source | EPA DSSTox | |
URL | https://comptox.epa.gov/dashboard/DTXSID10219181 | |
Description | DSSTox provides a high quality public chemistry resource for supporting improved predictive toxicology. | |
Molecular Weight |
203.06 g/mol | |
Source | PubChem | |
URL | https://pubchem.ncbi.nlm.nih.gov | |
Description | Data deposited in or computed by PubChem | |
CAS No. |
6912-28-3, 14281-77-7 | |
Record name | Manganese(II) bis(glycinate) | |
Source | ChemIDplus | |
URL | https://pubchem.ncbi.nlm.nih.gov/substance/?source=chemidplus&sourceid=0006912283 | |
Description | ChemIDplus is a free, web search system that provides access to the structure and nomenclature authority files used for the identification of chemical substances cited in National Library of Medicine (NLM) databases, including the TOXNET system. | |
Record name | Manganese glycinate | |
Source | ChemIDplus | |
URL | https://pubchem.ncbi.nlm.nih.gov/substance/?source=chemidplus&sourceid=0014281777 | |
Description | ChemIDplus is a free, web search system that provides access to the structure and nomenclature authority files used for the identification of chemical substances cited in National Library of Medicine (NLM) databases, including the TOXNET system. | |
Record name | Manganese(II) bis(glycinate) | |
Source | EPA DSSTox | |
URL | https://comptox.epa.gov/dashboard/DTXSID10219181 | |
Description | DSSTox provides a high quality public chemistry resource for supporting improved predictive toxicology. | |
Record name | Bis(glycinato)manganese | |
Source | European Chemicals Agency (ECHA) | |
URL | https://echa.europa.eu/information-on-chemicals | |
Description | The European Chemicals Agency (ECHA) is an agency of the European Union which is the driving force among regulatory authorities in implementing the EU's groundbreaking chemicals legislation for the benefit of human health and the environment as well as for innovation and competitiveness. | |
Explanation | Use of the information, documents and data from the ECHA website is subject to the terms and conditions of this Legal Notice, and subject to other binding limitations provided for under applicable law, the information, documents and data made available on the ECHA website may be reproduced, distributed and/or used, totally or in part, for non-commercial purposes provided that ECHA is acknowledged as the source: "Source: European Chemicals Agency, http://echa.europa.eu/". Such acknowledgement must be included in each copy of the material. ECHA permits and encourages organisations and individuals to create links to the ECHA website under the following cumulative conditions: Links can only be made to webpages that provide a link to the Legal Notice page. | |
Record name | MANGANESE GLYCINATE | |
Source | FDA Global Substance Registration System (GSRS) | |
URL | https://gsrs.ncats.nih.gov/ginas/app/beta/substances/B49L2D4K1D | |
Description | The FDA Global Substance Registration System (GSRS) enables the efficient and accurate exchange of information on what substances are in regulated products. Instead of relying on names, which vary across regulatory domains, countries, and regions, the GSRS knowledge base makes it possible for substances to be defined by standardized, scientific descriptions. | |
Explanation | Unless otherwise noted, the contents of the FDA website (www.fda.gov), both text and graphics, are not copyrighted. They are in the public domain and may be republished, reprinted and otherwise used freely by anyone without the need to obtain permission from FDA. Credit to the U.S. Food and Drug Administration as the source is appreciated but not required. | |
Retrosynthesis Analysis
AI-Powered Synthesis Planning: Our tool employs the Template_relevance Pistachio, Template_relevance Bkms_metabolic, Template_relevance Pistachio_ringbreaker, Template_relevance Reaxys, Template_relevance Reaxys_biocatalysis model, leveraging a vast database of chemical reactions to predict feasible synthetic routes.
One-Step Synthesis Focus: Specifically designed for one-step synthesis, it provides concise and direct routes for your target compounds, streamlining the synthesis process.
Accurate Predictions: Utilizing the extensive PISTACHIO, BKMS_METABOLIC, PISTACHIO_RINGBREAKER, REAXYS, REAXYS_BIOCATALYSIS database, our tool offers high-accuracy predictions, reflecting the latest in chemical research and data.
Strategy Settings
Precursor scoring | Relevance Heuristic |
---|---|
Min. plausibility | 0.01 |
Model | Template_relevance |
Template Set | Pistachio/Bkms_metabolic/Pistachio_ringbreaker/Reaxys/Reaxys_biocatalysis |
Top-N result to add to graph | 6 |
Feasible Synthetic Routes
Q1: How does Manganese Glycinate compare to Manganese Sulfate in terms of bioavailability and effects on laying hen performance?
A1: Research indicates that both this compound and Manganese Sulfate effectively enhance manganese levels in laying hens []. Both forms significantly improved feed intake, egg production, egg mass, and feed conversion ratio compared to a control group without manganese supplementation []. While both forms increased egg weight, no significant differences were observed in egg quality traits between the two manganese sources []. Importantly, this compound demonstrated superior bioavailability, leading to increased manganese deposition in the tibia, yolk, eggshells, serum, and liver compared to the control group []. This suggests that this compound might be a more efficient source of manganese supplementation for laying hens.
Q2: What is the industrial production process for this compound?
A2: One method involves reacting Manganese Sulfate Monohydrate with Sodium Hydroxide to produce Manganese Hydroxide []. After filtration and drying, the Manganese Hydroxide reacts with Sulfuric Acid, followed by the addition of Glycine []. The resulting solution is concentrated, and Acetone is added to precipitate solid this compound []. This process is recognized for its simplicity, safety, high yield, and minimal environmental impact [].
Q3: Can this compound be combined with other Glycine metal chelates, and what are the potential benefits?
A3: Yes, this compound can be combined with other Glycine metal chelates like Calcium Glycinate, Magnesium Glycinate, Zinc Glycinate, Ferrous Glycinate, Iron Glycinate, and Copper Glycinate []. Combining these chelates could offer a balanced approach to supplementing essential trace elements, addressing the body's requirements for Calcium, Magnesium, Zinc, Iron, Copper, and Manganese []. This method presents a simple and effective way to deliver multiple essential nutrients simultaneously.
Disclaimer and Information on In-Vitro Research Products
Please be aware that all articles and product information presented on BenchChem are intended solely for informational purposes. The products available for purchase on BenchChem are specifically designed for in-vitro studies, which are conducted outside of living organisms. In-vitro studies, derived from the Latin term "in glass," involve experiments performed in controlled laboratory settings using cells or tissues. It is important to note that these products are not categorized as medicines or drugs, and they have not received approval from the FDA for the prevention, treatment, or cure of any medical condition, ailment, or disease. We must emphasize that any form of bodily introduction of these products into humans or animals is strictly prohibited by law. It is essential to adhere to these guidelines to ensure compliance with legal and ethical standards in research and experimentation.