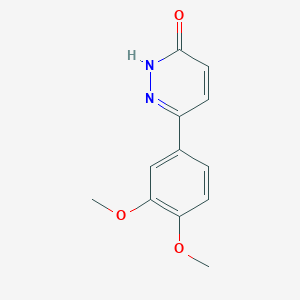
6-(3,4-dimethoxyphenyl)pyridazin-3(2H)-one
- Click on QUICK INQUIRY to receive a quote from our team of experts.
- With the quality product at a COMPETITIVE price, you can focus more on your research.
Overview
Description
6-(3,4-Dimethoxyphenyl)pyridazin-3(2H)-one is a heterocyclic compound with the molecular formula C12H12N2O3 It is characterized by a pyridazine ring substituted with a 3,4-dimethoxyphenyl group
Preparation Methods
Synthetic Routes and Reaction Conditions
The synthesis of 6-(3,4-dimethoxyphenyl)pyridazin-3(2H)-one typically involves the reaction of 3,4-dimethoxybenzaldehyde with hydrazine hydrate to form the corresponding hydrazone. This intermediate is then cyclized using an appropriate reagent, such as acetic anhydride, to yield the desired pyridazinone compound. The reaction conditions often include refluxing the mixture in a suitable solvent like ethanol or acetic acid.
Industrial Production Methods
Industrial production methods for this compound may involve similar synthetic routes but are optimized for large-scale production. This includes the use of continuous flow reactors and automated systems to ensure consistent quality and yield. The choice of solvents and reagents is also optimized to minimize costs and environmental impact.
Chemical Reactions Analysis
Types of Reactions
6-(3,4-Dimethoxyphenyl)pyridazin-3(2H)-one can undergo various chemical reactions, including:
Oxidation: The compound can be oxidized using agents like potassium permanganate or hydrogen peroxide to form corresponding oxidized derivatives.
Reduction: Reduction reactions can be carried out using reagents such as sodium borohydride or lithium aluminum hydride to yield reduced forms of the compound.
Substitution: The compound can undergo nucleophilic substitution reactions, particularly at the pyridazine ring, using reagents like alkyl halides or acyl chlorides.
Common Reagents and Conditions
Oxidation: Potassium permanganate in acidic or neutral conditions.
Reduction: Sodium borohydride in methanol or ethanol.
Substitution: Alkyl halides in the presence of a base like sodium hydroxide.
Major Products Formed
Oxidation: Oxidized derivatives with additional oxygen-containing functional groups.
Reduction: Reduced derivatives with fewer oxygen atoms.
Substitution: Substituted pyridazinone derivatives with various alkyl or acyl groups.
Scientific Research Applications
6-(3,4-Dimethoxyphenyl)pyridazin-3(2H)-one has several scientific research applications:
Chemistry: Used as a building block in the synthesis of more complex heterocyclic compounds.
Biology: Studied for its potential biological activities, including antimicrobial and antifungal properties.
Medicine: Investigated for its potential as a pharmaceutical intermediate in the development of new drugs.
Industry: Utilized in the production of specialty chemicals and materials with specific properties.
Mechanism of Action
The mechanism of action of 6-(3,4-dimethoxyphenyl)pyridazin-3(2H)-one involves its interaction with specific molecular targets and pathways. The compound may act by inhibiting certain enzymes or receptors, leading to its observed biological effects. Detailed studies on its molecular targets and pathways are ongoing to fully elucidate its mechanism of action.
Comparison with Similar Compounds
Similar Compounds
6-(3,4-Dimethylphenyl)pyridazin-3(2H)-one: Similar structure but with methyl groups instead of methoxy groups.
6-(3,4-Difluorophenyl)pyridazin-3(2H)-one: Contains fluorine atoms instead of methoxy groups.
6-(3,4-Dichlorophenyl)pyridazin-3(2H)-one: Contains chlorine atoms instead of methoxy groups.
Uniqueness
6-(3,4-Dimethoxyphenyl)pyridazin-3(2H)-one is unique due to the presence of methoxy groups, which can influence its chemical reactivity and biological activity. The methoxy groups can participate in hydrogen bonding and other interactions, potentially enhancing the compound’s effectiveness in various applications.
Properties
IUPAC Name |
3-(3,4-dimethoxyphenyl)-1H-pyridazin-6-one |
Source
|
---|---|---|
Source | PubChem | |
URL | https://pubchem.ncbi.nlm.nih.gov | |
Description | Data deposited in or computed by PubChem | |
InChI |
InChI=1S/C12H12N2O3/c1-16-10-5-3-8(7-11(10)17-2)9-4-6-12(15)14-13-9/h3-7H,1-2H3,(H,14,15) |
Source
|
Source | PubChem | |
URL | https://pubchem.ncbi.nlm.nih.gov | |
Description | Data deposited in or computed by PubChem | |
InChI Key |
ZHNHPPJSMFUHRS-UHFFFAOYSA-N |
Source
|
Source | PubChem | |
URL | https://pubchem.ncbi.nlm.nih.gov | |
Description | Data deposited in or computed by PubChem | |
Canonical SMILES |
COC1=C(C=C(C=C1)C2=NNC(=O)C=C2)OC |
Source
|
Source | PubChem | |
URL | https://pubchem.ncbi.nlm.nih.gov | |
Description | Data deposited in or computed by PubChem | |
Molecular Formula |
C12H12N2O3 |
Source
|
Source | PubChem | |
URL | https://pubchem.ncbi.nlm.nih.gov | |
Description | Data deposited in or computed by PubChem | |
Molecular Weight |
232.23 g/mol |
Source
|
Source | PubChem | |
URL | https://pubchem.ncbi.nlm.nih.gov | |
Description | Data deposited in or computed by PubChem | |
CAS No. |
55901-95-6 |
Source
|
Record name | 6-(3,4-dimethoxyphenyl)pyridazin-3-ol | |
Source | European Chemicals Agency (ECHA) | |
URL | https://echa.europa.eu/information-on-chemicals | |
Description | The European Chemicals Agency (ECHA) is an agency of the European Union which is the driving force among regulatory authorities in implementing the EU's groundbreaking chemicals legislation for the benefit of human health and the environment as well as for innovation and competitiveness. | |
Explanation | Use of the information, documents and data from the ECHA website is subject to the terms and conditions of this Legal Notice, and subject to other binding limitations provided for under applicable law, the information, documents and data made available on the ECHA website may be reproduced, distributed and/or used, totally or in part, for non-commercial purposes provided that ECHA is acknowledged as the source: "Source: European Chemicals Agency, http://echa.europa.eu/". Such acknowledgement must be included in each copy of the material. ECHA permits and encourages organisations and individuals to create links to the ECHA website under the following cumulative conditions: Links can only be made to webpages that provide a link to the Legal Notice page. | |
Disclaimer and Information on In-Vitro Research Products
Please be aware that all articles and product information presented on BenchChem are intended solely for informational purposes. The products available for purchase on BenchChem are specifically designed for in-vitro studies, which are conducted outside of living organisms. In-vitro studies, derived from the Latin term "in glass," involve experiments performed in controlled laboratory settings using cells or tissues. It is important to note that these products are not categorized as medicines or drugs, and they have not received approval from the FDA for the prevention, treatment, or cure of any medical condition, ailment, or disease. We must emphasize that any form of bodily introduction of these products into humans or animals is strictly prohibited by law. It is essential to adhere to these guidelines to ensure compliance with legal and ethical standards in research and experimentation.