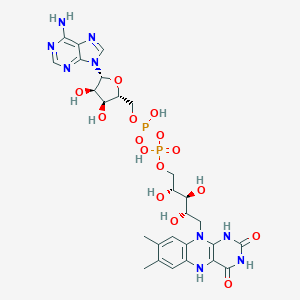
FADH2
- Click on QUICK INQUIRY to receive a quote from our team of experts.
- With the quality product at a COMPETITIVE price, you can focus more on your research.
Overview
Description
FADH2 is a redox cofactor derived from riboflavin (vitamin B2). It plays a crucial role in various biological processes, particularly in the oxidative metabolism of carbohydrates, amino acids, and fatty acids. The compound is essential for the functionality of flavoenzymes involved in mitochondrial electron transport and other cellular processes .
Scientific Research Applications
FADH2 has a wide range of scientific research applications:
Preparation Methods
Synthetic Routes and Reaction Conditions: FADH2 is synthesized from riboflavin through a series of enzymatic reactions. The primary synthetic route involves the phosphorylation of riboflavin to form flavin mononucleotide, followed by the adenylation of flavin mononucleotide to produce dihydroflavine-adenine dinucleotide .
Industrial Production Methods: Industrial production of dihydroflavine-adenine dinucleotide typically involves microbial fermentation processes. Specific strains of bacteria or yeast are genetically engineered to overproduce riboflavin, which is then converted to dihydroflavine-adenine dinucleotide through enzymatic pathways .
Chemical Reactions Analysis
Types of Reactions: FADH2 undergoes various chemical reactions, including oxidation, reduction, and substitution. It acts as an electron carrier in redox reactions, facilitating the transfer of electrons between different molecules .
Common Reagents and Conditions: Common reagents used in reactions involving dihydroflavine-adenine dinucleotide include oxidizing agents like oxygen and reducing agents such as nicotinamide adenine dinucleotide. The reactions typically occur under physiological conditions, with specific enzymes catalyzing the processes .
Major Products: The major products formed from reactions involving dihydroflavine-adenine dinucleotide include reduced or oxidized forms of the compound, depending on the specific reaction conditions and reagents used .
Mechanism of Action
FADH2 exerts its effects by acting as a redox cofactor in various enzymatic reactions. It facilitates the transfer of electrons between different molecules, thereby playing a crucial role in cellular respiration and energy production. The compound interacts with specific enzymes, such as dehydrogenases and oxidases, to catalyze redox reactions .
Comparison with Similar Compounds
- Flavin mononucleotide
- Nicotinamide adenine dinucleotide
- Nicotinamide adenine dinucleotide phosphate
Comparison: FADH2 is unique due to its conformational flexibility and ability to exist in multiple forms (stacked and unstacked). This versatility allows it to participate in a wide range of redox reactions and interact with various enzymes. In contrast, similar compounds like flavin mononucleotide and nicotinamide adenine dinucleotide have more rigid structures and specific roles in cellular processes .
Properties
CAS No. |
1910-41-4 |
---|---|
Molecular Formula |
C27H35N9O15P2 |
Molecular Weight |
787.6 g/mol |
IUPAC Name |
[[(2R,3S,4R,5R)-5-(6-aminopurin-9-yl)-3,4-dihydroxyoxolan-2-yl]methoxy-hydroxyphosphoryl] [(2R,3S,4S)-5-(7,8-dimethyl-2,4-dioxo-1,5-dihydrobenzo[g]pteridin-10-yl)-2,3,4-trihydroxypentyl] hydrogen phosphate |
InChI |
InChI=1S/C27H35N9O15P2/c1-10-3-12-13(4-11(10)2)35(24-18(32-12)25(42)34-27(43)33-24)5-14(37)19(39)15(38)6-48-52(44,45)51-53(46,47)49-7-16-20(40)21(41)26(50-16)36-9-31-17-22(28)29-8-30-23(17)36/h3-4,8-9,14-16,19-21,26,32,37-41H,5-7H2,1-2H3,(H,44,45)(H,46,47)(H2,28,29,30)(H2,33,34,42,43)/t14-,15+,16+,19-,20+,21+,26+/m0/s1 |
InChI Key |
YPZRHBJKEMOYQH-UYBVJOGSSA-N |
Isomeric SMILES |
CC1=CC2=C(C=C1C)N(C3=C(N2)C(=O)NC(=O)N3)C[C@@H]([C@@H]([C@@H](COP(=O)(O)OP(=O)(O)OC[C@@H]4[C@H]([C@H]([C@@H](O4)N5C=NC6=C(N=CN=C65)N)O)O)O)O)O |
SMILES |
CC1=CC2=C(C=C1C)N(C3=C(N2)C(=O)NC(=O)N3)CC(C(C(COP(=O)(O)OP(=O)(O)OCC4C(C(C(O4)N5C=NC6=C(N=CN=C65)N)O)O)O)O)O |
Canonical SMILES |
CC1=CC2=C(C=C1C)N(C3=C(N2)C(=O)NC(=O)N3)CC(C(C(COP(=O)(O)OP(=O)(O)OCC4C(C(C(O4)N5C=NC6=C(N=CN=C65)N)O)O)O)O)O |
1910-41-4 | |
physical_description |
Solid |
Synonyms |
1,5-dihydro-FAD FADH2 |
Origin of Product |
United States |
Retrosynthesis Analysis
AI-Powered Synthesis Planning: Our tool employs the Template_relevance Pistachio, Template_relevance Bkms_metabolic, Template_relevance Pistachio_ringbreaker, Template_relevance Reaxys, Template_relevance Reaxys_biocatalysis model, leveraging a vast database of chemical reactions to predict feasible synthetic routes.
One-Step Synthesis Focus: Specifically designed for one-step synthesis, it provides concise and direct routes for your target compounds, streamlining the synthesis process.
Accurate Predictions: Utilizing the extensive PISTACHIO, BKMS_METABOLIC, PISTACHIO_RINGBREAKER, REAXYS, REAXYS_BIOCATALYSIS database, our tool offers high-accuracy predictions, reflecting the latest in chemical research and data.
Strategy Settings
Precursor scoring | Relevance Heuristic |
---|---|
Min. plausibility | 0.01 |
Model | Template_relevance |
Template Set | Pistachio/Bkms_metabolic/Pistachio_ringbreaker/Reaxys/Reaxys_biocatalysis |
Top-N result to add to graph | 6 |
Feasible Synthetic Routes
Disclaimer and Information on In-Vitro Research Products
Please be aware that all articles and product information presented on BenchChem are intended solely for informational purposes. The products available for purchase on BenchChem are specifically designed for in-vitro studies, which are conducted outside of living organisms. In-vitro studies, derived from the Latin term "in glass," involve experiments performed in controlled laboratory settings using cells or tissues. It is important to note that these products are not categorized as medicines or drugs, and they have not received approval from the FDA for the prevention, treatment, or cure of any medical condition, ailment, or disease. We must emphasize that any form of bodily introduction of these products into humans or animals is strictly prohibited by law. It is essential to adhere to these guidelines to ensure compliance with legal and ethical standards in research and experimentation.