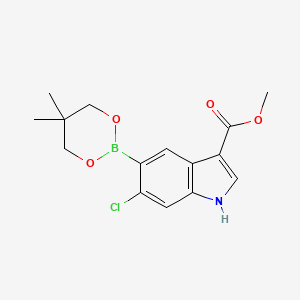
methyl 6-chloro-5-(5,5-dimethyl-1,3,2-dioxaborinan-2-yl)-1H-indole-3-carboxylate
- Click on QUICK INQUIRY to receive a quote from our team of experts.
- With the quality product at a COMPETITIVE price, you can focus more on your research.
Overview
Description
Methyl 6-chloro-5-(5,5-dimethyl-1,3,2-dioxaborinan-2-yl)-1H-indole-3-carboxylate is a complex organic compound that features a unique combination of functional groups, including a boronate ester, a chloro substituent, and an indole core
Preparation Methods
The synthesis of methyl 6-chloro-5-(5,5-dimethyl-1,3,2-dioxaborinan-2-yl)-1H-indole-3-carboxylate typically involves multi-step organic reactions. One common synthetic route includes the following steps:
Formation of the Indole Core: The indole core can be synthesized through Fischer indole synthesis or other methods involving cyclization of appropriate precursors.
Introduction of the Chloro Substituent: Chlorination of the indole core can be achieved using reagents such as thionyl chloride or phosphorus pentachloride.
Boronate Ester Formation: The boronate ester group can be introduced through a reaction with boronic acid or its derivatives under suitable conditions.
Esterification: The final step involves esterification to introduce the methyl ester group, typically using methanol and an acid catalyst.
Industrial production methods may involve optimization of these steps to enhance yield and purity, as well as the use of continuous flow reactors for large-scale synthesis.
Chemical Reactions Analysis
Methyl 6-chloro-5-(5,5-dimethyl-1,3,2-dioxaborinan-2-yl)-1H-indole-3-carboxylate undergoes various types of chemical reactions, including:
Oxidation: The compound can be oxidized using oxidizing agents such as hydrogen peroxide or potassium permanganate, leading to the formation of corresponding oxidized products.
Reduction: Reduction reactions can be carried out using reducing agents like lithium aluminum hydride or sodium borohydride, resulting in the reduction of specific functional groups.
Substitution: The chloro substituent can be replaced through nucleophilic substitution reactions using nucleophiles such as amines or thiols.
Coupling Reactions: The boronate ester group allows for Suzuki-Miyaura coupling reactions with aryl halides to form biaryl compounds.
Common reagents and conditions used in these reactions include organic solvents (e.g., dichloromethane, tetrahydrofuran), catalysts (e.g., palladium catalysts for coupling reactions), and temperature control.
Scientific Research Applications
Methyl 6-chloro-5-(5,5-dimethyl-1,3,2-dioxaborinan-2-yl)-1H-indole-3-carboxylate has a wide range of scientific research applications:
Organic Synthesis: It serves as a versatile building block for the synthesis of complex organic molecules, particularly in the development of pharmaceuticals and agrochemicals.
Medicinal Chemistry: The compound is investigated for its potential as a pharmacophore in drug design, targeting specific biological pathways and receptors.
Material Science: It is used in the synthesis of novel materials with unique electronic and optical properties, which are of interest in the development of sensors and electronic devices.
Biological Studies: The compound’s interactions with biological macromolecules are studied to understand its potential therapeutic effects and mechanisms of action.
Mechanism of Action
The mechanism of action of methyl 6-chloro-5-(5,5-dimethyl-1,3,2-dioxaborinan-2-yl)-1H-indole-3-carboxylate involves its interaction with specific molecular targets and pathways. The boronate ester group can form reversible covalent bonds with diols and other nucleophiles, which is exploited in the design of enzyme inhibitors and sensors. The indole core may interact with various receptors and enzymes, modulating their activity and leading to biological effects.
Comparison with Similar Compounds
Methyl 6-chloro-5-(5,5-dimethyl-1,3,2-dioxaborinan-2-yl)-1H-indole-3-carboxylate can be compared with other similar compounds, such as:
6-Chloroindole Derivatives: These compounds share the chloroindole core but differ in the substituents attached to the indole ring.
Boronate Ester Compounds: These compounds contain the boronate ester group but may have different aromatic or heterocyclic cores.
Indole-3-Carboxylate Esters: These compounds have the indole-3-carboxylate structure but vary in the substituents on the indole ring and ester group.
Properties
IUPAC Name |
methyl 6-chloro-5-(5,5-dimethyl-1,3,2-dioxaborinan-2-yl)-1H-indole-3-carboxylate |
Source
|
---|---|---|
Source | PubChem | |
URL | https://pubchem.ncbi.nlm.nih.gov | |
Description | Data deposited in or computed by PubChem | |
InChI |
InChI=1S/C15H17BClNO4/c1-15(2)7-21-16(22-8-15)11-4-9-10(14(19)20-3)6-18-13(9)5-12(11)17/h4-6,18H,7-8H2,1-3H3 |
Source
|
Source | PubChem | |
URL | https://pubchem.ncbi.nlm.nih.gov | |
Description | Data deposited in or computed by PubChem | |
InChI Key |
PTICAZXUYAIOSL-UHFFFAOYSA-N |
Source
|
Source | PubChem | |
URL | https://pubchem.ncbi.nlm.nih.gov | |
Description | Data deposited in or computed by PubChem | |
Canonical SMILES |
B1(OCC(CO1)(C)C)C2=CC3=C(C=C2Cl)NC=C3C(=O)OC |
Source
|
Source | PubChem | |
URL | https://pubchem.ncbi.nlm.nih.gov | |
Description | Data deposited in or computed by PubChem | |
Molecular Formula |
C15H17BClNO4 |
Source
|
Source | PubChem | |
URL | https://pubchem.ncbi.nlm.nih.gov | |
Description | Data deposited in or computed by PubChem | |
Molecular Weight |
321.6 g/mol |
Source
|
Source | PubChem | |
URL | https://pubchem.ncbi.nlm.nih.gov | |
Description | Data deposited in or computed by PubChem | |
Disclaimer and Information on In-Vitro Research Products
Please be aware that all articles and product information presented on BenchChem are intended solely for informational purposes. The products available for purchase on BenchChem are specifically designed for in-vitro studies, which are conducted outside of living organisms. In-vitro studies, derived from the Latin term "in glass," involve experiments performed in controlled laboratory settings using cells or tissues. It is important to note that these products are not categorized as medicines or drugs, and they have not received approval from the FDA for the prevention, treatment, or cure of any medical condition, ailment, or disease. We must emphasize that any form of bodily introduction of these products into humans or animals is strictly prohibited by law. It is essential to adhere to these guidelines to ensure compliance with legal and ethical standards in research and experimentation.