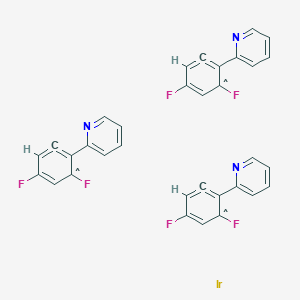
Ir(dFppy)3
- Click on QUICK INQUIRY to receive a quote from our team of experts.
- With the quality product at a COMPETITIVE price, you can focus more on your research.
Overview
Description
Ir(dFppy)<SUB>3</SUB> is a photocatalyst that readily facilitates the decarboxylative arylation of α amino acids using visible light .
Synthesis Analysis
The synthesis of Ir(dFppy)<SUB>3</SUB> involves a C–F bond activation reaction of a chloro-bridged iridium (III) dimer in the presence of sodium methoxide . The empirical formula is C33H18F6IrN3 and the molecular weight is 762.72 .
Molecular Structure Analysis
The molecular structure of Ir(dFppy)<SUB>3</SUB> is complex and involves the coordination of iridium with three dFppy ligands . The SMILES string for this compound is FC1=CC([Ir]C2=C(C3=NC=CC=C3)C(F)=CC(F)=C2)=C(C4=NC=CC=C4)C(F)=C1.FC5=CC(F)=CC=C5C6=NC=CC=C6 .
Chemical Reactions Analysis
Ir(dFppy)<SUB>3</SUB> has been used in various chemical reactions. It facilitates the decarboxylative arylation of α amino acids using visible light . It has also been used in the light-driven CO2 reduction , and in the annulation-alkynylation of alkenes reaction .
Physical And Chemical Properties Analysis
Ir(dFppy)<SUB>3</SUB> is a powder or crystal with a melting point of 401°C . It has a high triplet state energy and its excited state redox potentials are comparatively diminished when compared to Ir(ppy)<SUB>3</SUB> .
Scientific Research Applications
Photocatalysis and Organic Synthesis
Ir(dFppy)3 serves as an efficient photocatalyst for various organic transformations. Its high triplet state energy (60 kcal/mol) enables it to facilitate photochemical reactions. Notably, it promotes the decarboxylative arylation of α-amino acids using visible light. Researchers have employed Ir(dFppy)3 in photoreactors, such as the Penn PhD and SynLED 2.0, to achieve selective C–C bond formation .
Light-Emitting Devices (OLEDs)
The luminescent properties of Ir(dFppy)3 make it a valuable component in organic light-emitting diodes (OLEDs). By incorporating this compound into OLED structures, scientists enhance device efficiency and color purity. Ir(dFppy)3 contributes to green and blue emission, leading to brighter and more energy-efficient displays .
Single Electron Transfer Reactions
Despite its common use in energy transfer reactions, Ir(dFppy)3 remains effective in single electron transfer reactions. For instance, it acts as an optimal catalyst for annulation-alkynylation of alkenes. Its unique combination of high triplet state energy and diminished excited state redox potentials sets it apart from other iridium complexes .
Mechanism of Action
Target of Action
The primary target of Ir(dFppy)<SUB>3</SUB> is α-amino acids . The compound acts as a photocatalyst that facilitates the decarboxylative arylation of these amino acids .
Mode of Action
Ir(dFppy)<SUB>3</SUB> interacts with its targets through a process known as photocatalysis . This process involves the absorption of light by the photocatalyst, which then transfers energy to the α-amino acids . The high triplet state energy and low redox potential of Ir(dFppy)<SUB>3</SUB> prevent undesired redox chemistry from occurring on the electron-rich indole and indoline ring systems .
Biochemical Pathways
The action of Ir(dFppy)<SUB>3</SUB> affects the decarboxylation pathway of α-amino acids . This leads to the formation of arylated products, a process that is facilitated by the energy transferred from the photocatalyst .
Result of Action
The result of the action of Ir(dFppy)<SUB>3</SUB> is the decarboxylative arylation of α-amino acids . This process converts these amino acids into arylated products .
Action Environment
The action of Ir(dFppy)<SUB>3</SUB> is influenced by environmental factors such as light. Specifically, the compound requires visible light to act as a photocatalyst . The use of photoreactors can enhance the efficacy and stability of its action .
Safety and Hazards
Future Directions
properties
{ "Design of the Synthesis Pathway": "The synthesis pathway for Ir(dFppy)3 involves the reaction of iridium(III) chloride hydrate with 2-(2,4-difluorophenyl)pyridine (dFppy) in the presence of a base and a reducing agent.", "Starting Materials": [ "Iridium(III) chloride hydrate", "2-(2,4-difluorophenyl)pyridine (dFppy)", "Base (e.g. potassium carbonate)", "Reducing agent (e.g. sodium borohydride)" ], "Reaction": [ "1. Dissolve iridium(III) chloride hydrate in a suitable solvent (e.g. ethanol) to form a solution.", "2. Add the base (e.g. potassium carbonate) to the solution and stir until dissolved.", "3. Add the reducing agent (e.g. sodium borohydride) to the solution and stir until dissolved.", "4. Add the 2-(2,4-difluorophenyl)pyridine (dFppy) to the solution and stir for several hours at room temperature.", "5. Heat the solution to reflux for several hours to complete the reaction.", "6. Cool the solution and filter the resulting solid.", "7. Wash the solid with a suitable solvent (e.g. ethanol) and dry under vacuum to obtain the final product, Ir(dFppy)3." ] } | |
CAS RN |
387859-70-3 |
Molecular Formula |
C33H18F6IrN3 |
Molecular Weight |
762.735 |
InChI |
InChI=1S/3C11H6F2N.Ir/c3*12-8-4-5-9(10(13)7-8)11-3-1-2-6-14-11;/h3*1-4,6-7H; |
InChI Key |
GFSFWLGQTIKUOK-UHFFFAOYSA-N |
SMILES |
C1=CC=NC(=C1)C2=C=CC(=C[C]2F)F.C1=CC=NC(=C1)C2=C=CC(=C[C]2F)F.C1=CC=NC(=C1)C2=C=CC(=C[C]2F)F.[Ir] |
solubility |
not available |
Origin of Product |
United States |
Q & A
Q1: What makes Ir(dFppy)3 a suitable photocatalyst for CO2 reduction?
A1: Ir(dFppy)3 exhibits several characteristics that make it a promising photocatalyst for CO2 reduction. [, , , ]. Firstly, it absorbs light in the visible region of the electromagnetic spectrum, allowing it to utilize sunlight as an energy source []. Secondly, it possesses a long-lived excited state, which is crucial for efficient electron transfer processes involved in photocatalysis []. Finally, its photophysical and electrochemical properties can be fine-tuned through ligand modification, as demonstrated by the varying performances of closely related copper(I) photosensitizers [].
Q2: How does the deuteriation of ligands impact the quantum efficiency of Ir(III) complexes like Ir(dFppy)3?
A2: Deuteriation of ligands in Ir(III) complexes like Ir(dFppy)3 can significantly enhance their intrinsic quantum efficiency []. This enhancement stems from the suppression of non-radiative decay processes, which compete with radiative decay (luminescence) []. Due to the higher mass of deuterium compared to hydrogen, deuteration leads to a decrease in the amplitude and frequency of vibrational modes within the molecule []. This, in turn, reduces the efficiency of non-radiative decay pathways, leading to an increase in the quantum yield of luminescence [].
Q3: Can Ir(dFppy)3 be incorporated into zeolites, and what are the implications for its luminescent properties?
A3: Yes, Ir(dFppy)3 can be successfully synthesized and encapsulated within the supercages of faujasite-type zeolites using a microwave-assisted ship-in-a-bottle method []. Interestingly, Ir(dFppy)3 encapsulated in zeolites exhibits a relatively high luminescence quantum yield of 14.34% []. This demonstrates the potential of using zeolites as hosts for luminescent Ir(III) complexes, offering a route to new hybrid materials with tailored photophysical properties [].
Q4: How do structural variations in closely related copper(I) photosensitizers, compared to Ir(dFppy)3, affect their photocatalytic activity?
A4: Research on structurally related copper(I) photosensitizers highlights the significant impact of even minor structural modifications on photophysical and photocatalytic properties []. For instance, changing the position of substituents on the phenanthroline ligand can drastically alter the absorption coefficient, emission lifetime, and ultimately, the photocatalytic efficiency for water splitting and CO2 reduction []. This emphasizes the importance of precise molecular design in optimizing photocatalysts for specific applications.
Q5: Can Ir(dFppy)3 be used in conjunction with other catalysts to enhance CO2 reduction?
A5: Yes, Ir(dFppy)3 can be effectively combined with other catalysts, such as dinuclear rhenium complexes, to enhance CO2 reduction to CO []. In these systems, Ir(dFppy)3 acts as a photosensitizer, absorbing light energy and transferring it to the rhenium complex, which then catalyzes the CO2 reduction reaction []. This synergistic effect highlights the potential of developing hybrid photocatalytic systems with improved performance compared to individual components.
Disclaimer and Information on In-Vitro Research Products
Please be aware that all articles and product information presented on BenchChem are intended solely for informational purposes. The products available for purchase on BenchChem are specifically designed for in-vitro studies, which are conducted outside of living organisms. In-vitro studies, derived from the Latin term "in glass," involve experiments performed in controlled laboratory settings using cells or tissues. It is important to note that these products are not categorized as medicines or drugs, and they have not received approval from the FDA for the prevention, treatment, or cure of any medical condition, ailment, or disease. We must emphasize that any form of bodily introduction of these products into humans or animals is strictly prohibited by law. It is essential to adhere to these guidelines to ensure compliance with legal and ethical standards in research and experimentation.