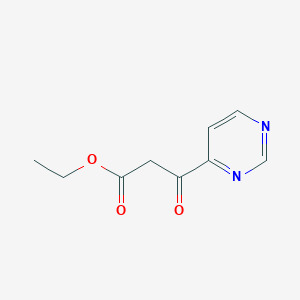
Ethyl 3-oxo-3-(pyrimidin-4-yl)propanoate
Overview
Description
Ethyl 3-oxo-3-(pyrimidin-4-yl)propanoate (CAS: 64210-67-9) is a β-keto ester derivative with the molecular formula C₉H₁₀N₂O₃ and a molecular weight of 194.19 g/mol . Structurally, it features a pyrimidin-4-yl group linked to a 3-oxopropanoate ethyl ester backbone. Pyrimidine, a six-membered heterocycle with two nitrogen atoms at positions 1 and 3, confers distinct electronic properties that influence reactivity and intermolecular interactions.
This compound serves as a critical intermediate in medicinal chemistry, particularly in synthesizing heterocyclic scaffolds for drug discovery. For example, it is utilized in Biginelli-like reactions to generate pyrimidinone derivatives, which are explored for antimicrobial and anticancer activities . Its synthesis typically involves condensation reactions between ethyl acetoacetate and pyrimidine derivatives under basic conditions, though detailed protocols are proprietary or context-dependent .
Preparation Methods
Claisen Condensation: Primary Laboratory-Scale Synthesis
The Claisen condensation represents the most widely documented method for synthesizing ethyl 3-oxo-3-(pyrimidin-4-yl)propanoate. This one-step approach involves the nucleophilic acyl substitution between ethyl acetoacetate and a pyrimidine-4-carbonyl derivative, typically pyrimidine-4-carbonyl chloride or pyrimidine-4-carboxylic acid activated in situ.
Reaction Mechanism and Conditions
The reaction proceeds via deprotonation of ethyl acetoacetate by a strong base (e.g., sodium ethoxide or lithium hexamethyldisilazane) to form an enolate intermediate. This enolate attacks the electrophilic carbonyl carbon of the pyrimidine derivative, followed by elimination of a leaving group (e.g., chloride or hydroxyl) to yield the β-keto ester. Critical parameters include:
- Temperature : Maintained at -40°C to minimize side reactions such as self-condensation of ethyl acetoacetate.
- Solvent : Tetrahydrofuran (THF) or anhydrous diethyl ether, chosen for their ability to dissolve both polar and nonpolar reactants.
- Atmosphere : Conducted under inert gas (N₂ or Ar) to prevent oxidation of intermediates.
Table 1: Standard Claisen Condensation Protocol
Two-Step Synthesis via Propionic Acid Intermediate
An alternative route involves synthesizing 3-oxo-3-(pyrimidin-4-yl)propionic acid followed by esterification with ethanol. This method is preferred when high-purity intermediates are required for downstream applications.
Step 1: Acid Formation
Pyrimidine-4-carboxylic acid undergoes condensation with ethyl acetoacetate under acidic conditions (H₂SO₄ or p-toluenesulfonic acid) to form the β-keto acid. The reaction is typically refluxed in toluene for 8–12 hours, achieving yields of 68–72% after recrystallization.
Step 2: Esterification
The isolated β-keto acid is esterified using ethanol in the presence of concentrated sulfuric acid as a catalyst. Key conditions include:
- Molar Ratio : 1:5 (acid:ethanol) to drive equilibrium toward ester formation.
- Temperature : Reflux at 78°C for 24 hours.
- Purification : Neutralization with NaHCO₃, followed by column chromatography (silica gel, ethyl acetate/hexane).
Table 2: Two-Step Synthesis Comparative Metrics
Metric | Claisen Condensation | Two-Step Method |
---|---|---|
Total Yield | ~65% (estimated) | 60–65% |
Purity (HPLC) | ≥94% | ≥97% |
Scalability | High | Moderate |
Equipment Complexity | Low | High |
Industrial-Scale Production
Industrial protocols adapt the Claisen condensation for continuous manufacturing, addressing challenges in heat management and mixing efficiency.
Continuous Flow Reactor Design
- Reactor Type : Tubular flow reactor with static mixers ensures rapid heat dissipation and homogeneous mixing.
- Residence Time : 30–45 minutes at -20°C, significantly shorter than batch processes.
- Throughput : Capable of producing 50–100 kg/day in pilot-scale systems.
Purification Infrastructure
- Liquid-Liquid Extraction : Countercurrent centrifugal extractors separate the product from aqueous waste streams.
- Drying : Spray dryers or thin-film evaporators remove residual solvents to <0.1% w/w.
Emerging Methodologies and Innovations
Enzymatic Catalysis
Recent studies explore lipase-catalyzed esterification as a green chemistry alternative. Candida antarctica lipase B (CAL-B) immobilized on mesoporous silica demonstrates 58% conversion at 37°C in solvent-free conditions, though reaction times extend to 72 hours.
Microwave-Assisted Synthesis
Microwave irradiation (150 W, 80°C) reduces Claisen condensation time to 20 minutes, achieving comparable yields to conventional heating. This method remains experimental but shows promise for rapid small-batch production.
Analytical Characterization
Post-synthesis quality control employs multiple techniques:
Table 3: Characterization Data
Chemical Reactions Analysis
Types of Reactions
Ethyl 3-oxo-3-(pyrimidin-4-yl)propanoate undergoes various chemical reactions, including:
Oxidation: This compound can be oxidized to form carboxylic acids or other oxidized derivatives.
Reduction: Reduction reactions can convert the keto group to an alcohol group.
Substitution: The compound can undergo nucleophilic substitution reactions, particularly at the carbonyl carbon.
Condensation: It can participate in condensation reactions to form larger molecules.
Common Reagents and Conditions
Oxidation: Common oxidizing agents include potassium permanganate (KMnO4) and chromium trioxide (CrO3).
Reduction: Reducing agents such as sodium borohydride (NaBH4) or lithium aluminum hydride (LiAlH4) are used.
Substitution: Nucleophiles like amines or thiols can be used in substitution reactions.
Condensation: Aldehydes or ketones can be used in condensation reactions under acidic or basic conditions.
Major Products
Oxidation: Carboxylic acids or esters.
Reduction: Alcohols.
Substitution: Various substituted derivatives depending on the nucleophile used.
Condensation: β-dicarbonyl compounds or other larger molecules.
Scientific Research Applications
Organic Synthesis
Ethyl 3-oxo-3-(pyrimidin-4-yl)propanoate serves as a versatile building block in the synthesis of various heterocyclic compounds. Its ability to undergo substitution and condensation reactions makes it suitable for creating complex organic molecules used in pharmaceuticals and agrochemicals .
Research indicates that this compound exhibits potential biological activities, including:
- Antimicrobial Properties : Studies have shown that derivatives of this compound possess antimicrobial effects against various pathogens, making them candidates for developing new antibiotics.
- Anticancer Activity : Preliminary investigations suggest that it may inhibit enzymes involved in DNA synthesis or repair, presenting opportunities for its use as an anticancer agent.
Medicinal Chemistry
The compound is being explored for its potential role in drug development. Its structure allows it to act as a precursor for synthesizing pharmaceuticals targeting specific diseases. For instance, modifications to its structure can lead to compounds with enhanced therapeutic efficacy or reduced side effects .
Case Study 1: Antimicrobial Activity
In a study published in the Asian Journal of Chemistry, researchers synthesized various derivatives of this compound and tested their antimicrobial activity against several bacterial strains. The results indicated that certain derivatives exhibited significant inhibition zones, suggesting their potential as new antimicrobial agents .
Case Study 2: Anticancer Research
A research team investigated the anticancer properties of this compound in vitro. The compound was found to induce apoptosis in cancer cell lines by inhibiting specific kinases involved in cell proliferation. This study highlights its potential application in cancer therapy development .
Mechanism of Action
The mechanism of action of Ethyl 3-oxo-3-(pyrimidin-4-yl)propanoate involves its interaction with specific molecular targets and pathways. The compound can act as an enzyme inhibitor or modulator, affecting various biochemical pathways. For example, it may inhibit enzymes involved in DNA synthesis or repair, leading to its potential use as an anticancer agent . The exact molecular targets and pathways depend on the specific application and the biological system being studied.
Comparison with Similar Compounds
The structural and functional analogs of ethyl 3-oxo-3-(pyrimidin-4-yl)propanoate vary in substituents at the β-keto ester’s 3-position. Below is a comparative analysis of key derivatives:
Table 1: Comparative Analysis of this compound and Analogous Compounds
Key Observations:
Substituent Electronic Effects :
- Pyrimidin-4-yl and pyridin-4-yl groups differ in nitrogen atom positioning, altering electronic density. Pyrimidine’s dual nitrogen atoms increase electron deficiency, enhancing reactivity in nucleophilic additions compared to pyridine derivatives .
- The 2-thienyl substituent (in ) enables high stereoselectivity in enzymatic reductions due to sulfur’s polarizability, making it valuable for chiral intermediate synthesis .
Steric and Solubility Considerations :
- Cyclopropyl-substituted analogs () exhibit increased steric hindrance, limiting accessibility in reactions but improving metabolic stability in drug candidates .
- Trifluoromethylphenyl derivatives () enhance lipophilicity and bioavailability, critical for blood-brain barrier penetration in CNS-targeting drugs .
Synthetic Utility :
- Pyrimidin-4-yl and pyridin-4-yl derivatives are pivotal in multicomponent reactions (e.g., Biginelli, Hantzsch) to construct polyheterocyclic systems .
- Nitro-substituted pyridinyl analogs () show moderate yields (45–50%) due to competing side reactions, underscoring the need for optimized conditions .
Biological Activity
Ethyl 3-oxo-3-(pyrimidin-4-yl)propanoate is a compound of significant interest due to its potential biological activities, particularly in medicinal chemistry. This article reviews the synthesis, biological properties, and potential applications of this compound, drawing from diverse sources.
Synthesis
The synthesis of this compound typically involves the reaction of ethyl isonicotinate with ethyl acetate in the presence of sodium ethoxide. The process includes refluxing the reactants and subsequent hydrolysis to yield the desired product. The compound can exist in both keto and enol forms, with the enolic form being predominant in certain solvents .
Antimicrobial Activity
This compound has shown promising antimicrobial properties. Studies have indicated that derivatives of pyrimidine compounds exhibit significant activity against various bacterial strains. For instance, a related compound demonstrated effective inhibition of Gram-positive and Gram-negative bacteria, suggesting that this compound may possess similar antimicrobial capabilities .
Antioxidant Activity
Research indicates that compounds containing pyrimidine rings often exhibit antioxidant properties. The antioxidant activity is typically evaluated using assays such as DPPH and ABTS, where higher radical scavenging activity correlates with increased potential for therapeutic applications. Preliminary findings suggest that ethyl derivatives can effectively scavenge free radicals, thereby contributing to their biological efficacy .
Enzyme Inhibition
This compound may act as an inhibitor for specific enzymes involved in metabolic pathways. For example, studies on similar pyrimidine derivatives have highlighted their ability to inhibit enzymes linked to cancer metabolism, such as mutant isocitrate dehydrogenase (IDH). This suggests that this compound could be explored for its potential role in cancer therapeutics .
Case Studies
- Antimicrobial Efficacy : A study focusing on pyrimidine derivatives highlighted the effectiveness of this compound against Staphylococcus aureus and Escherichia coli. The compound exhibited a minimum inhibitory concentration (MIC) comparable to standard antibiotics, indicating its potential as an antimicrobial agent .
- Antioxidant Assessment : In a comparative analysis of various pyrimidine derivatives, this compound was found to have an IC50 value of 150 µM in DPPH assays, demonstrating significant antioxidant activity that could be beneficial in preventing oxidative stress-related diseases .
Research Findings
Recent studies emphasize the need for further research into the pharmacokinetics and toxicity profiles of this compound. Preliminary data suggest favorable characteristics such as low permeability across the blood-brain barrier (BBB), which may influence its application in central nervous system disorders . Additionally, toxicity assessments indicate that this compound does not significantly inhibit major cytochrome P450 enzymes, suggesting a lower risk for drug-drug interactions .
Q & A
Q. Basic: What are the optimal synthetic routes for Ethyl 3-oxo-3-(pyrimidin-4-yl)propanoate, and how can yield be maximized?
Answer:
The synthesis typically involves esterification of 3-oxo-3-(pyrimidin-4-yl)propanoic acid with ethanol under reflux conditions using a strong acid catalyst (e.g., sulfuric acid). Evidence from analogous compounds suggests yields can reach ~86% when using excess ethanol and controlled reflux times (e.g., 6–8 hours) to drive the reaction to completion . Alternative routes, such as transesterification or nucleophilic substitution, may yield lower outputs (~42%) due to competing side reactions, necessitating purification via column chromatography .
Q. Basic: Which analytical techniques are critical for confirming the molecular structure of this compound?
Answer:
Nuclear Magnetic Resonance (NMR) spectroscopy is essential for verifying the pyrimidinyl and ester moieties, with -NMR peaks expected at δ ~8.8 ppm (pyrimidine protons) and δ ~4.2 ppm (ethyl ester group). Infrared (IR) spectroscopy confirms the carbonyl stretch (~1720 cm) and pyrimidine ring vibrations (~1600 cm). High-resolution mass spectrometry (HRMS) provides molecular weight validation (theoretical: 193.20 g/mol) .
Q. Advanced: How can X-ray crystallography resolve structural ambiguities in derivatives of this compound?
Answer:
Single-crystal X-ray diffraction using programs like SHELXL or ORTEP-3 can determine bond lengths, angles, and torsion angles, critical for distinguishing keto-enol tautomerism or conformational isomers. For example, the C=O bond length in the oxo group (~1.21 Å) and pyrimidine ring planarity can confirm the absence of unexpected distortions. Crystallographic data should be refined with a resolution < 0.8 Å to ensure accuracy .
Q. Advanced: What methodological strategies address low stereoselectivity in bioreductions of the 3-oxo group?
Answer:
Enzymatic reduction using ketoreductases (e.g., ChKRED12) under optimized pH (7.0–7.5) and cofactor conditions (NADPH, 1–2 mM) can achieve >90% enantiomeric excess (ee). Substrate engineering (e.g., protecting the pyrimidine nitrogen) may reduce steric hindrance and improve enzyme-substrate binding. Kinetic studies (e.g., and ) should guide process scaling .
Q. Advanced: How do QSAR models predict the biological activity of pyrimidine-containing analogs?
Answer:
Quantitative Structure-Activity Relationship (QSAR) models use descriptors like logP (lipophilicity), polar surface area, and H-bond acceptor counts. For example, derivatives with logP < 2.5 may exhibit enhanced membrane permeability, while electron-withdrawing groups on the pyrimidine ring (e.g., -Cl) can increase binding affinity to kinase targets. Computational tools (e.g., Schrödinger Suite) validate these predictions via docking simulations .
Q. Basic: What are common functionalization reactions of the 3-oxo group in this compound?
Answer:
The oxo group undergoes:
- Claisen condensation with esters to form β-diketo derivatives.
- Reduction to 3-hydroxypropanoate using NaBH/CeCl (Luche conditions).
- Nucleophilic substitution with hydrazines to form pyrazole analogs. Reaction conditions (e.g., anhydrous solvents, 0–5°C) minimize side reactions like over-reduction .
Q. Advanced: How can discrepancies in synthetic yields between routes be systematically analyzed?
Answer:
Design a Design of Experiments (DoE) approach varying parameters (temperature, catalyst loading, solvent polarity). For example, a central composite design can identify critical factors (e.g., ethanol purity) impacting yield. Reaction monitoring via TLC or in situ FTIR helps detect intermediates or byproducts (e.g., unreacted acid) that reduce efficiency .
Q. Advanced: What computational tools assist in predicting the compound’s reactivity in complex matrices?
Answer:
Density Functional Theory (DFT) calculations (e.g., B3LYP/6-31G**) model transition states for reactions like ester hydrolysis. Molecular dynamics simulations (e.g., GROMACS) predict solvation effects in biological systems. These tools guide the design of stable derivatives for in vitro assays .
Q. Basic: What precautions are necessary for handling and storing this compound?
Answer:
Store under inert atmosphere (N or Ar) at room temperature to prevent ester hydrolysis. Use anhydrous solvents (e.g., THF, DMF) for reactions. Safety protocols include PPE (gloves, goggles) due to potential skin/eye irritation (H315, H319) .
Q. Advanced: How can structural analogs be designed to enhance binding to pyrimidine-specific enzymes?
Answer:
Introduce bioisosteric replacements (e.g., replacing pyrimidine with 1,3,5-triazine) while maintaining hydrogen-bonding motifs. Conformational restriction via cyclopropane rings (e.g., trans-2-phenylcyclopropyl groups) can mimic transition states, as seen in indole carboxylate derivatives .
Properties
IUPAC Name |
ethyl 3-oxo-3-pyrimidin-4-ylpropanoate | |
---|---|---|
Source | PubChem | |
URL | https://pubchem.ncbi.nlm.nih.gov | |
Description | Data deposited in or computed by PubChem | |
InChI |
InChI=1S/C9H10N2O3/c1-2-14-9(13)5-8(12)7-3-4-10-6-11-7/h3-4,6H,2,5H2,1H3 | |
Source | PubChem | |
URL | https://pubchem.ncbi.nlm.nih.gov | |
Description | Data deposited in or computed by PubChem | |
InChI Key |
IGRUUQSUGJLZCM-UHFFFAOYSA-N | |
Source | PubChem | |
URL | https://pubchem.ncbi.nlm.nih.gov | |
Description | Data deposited in or computed by PubChem | |
Canonical SMILES |
CCOC(=O)CC(=O)C1=NC=NC=C1 | |
Source | PubChem | |
URL | https://pubchem.ncbi.nlm.nih.gov | |
Description | Data deposited in or computed by PubChem | |
Molecular Formula |
C9H10N2O3 | |
Source | PubChem | |
URL | https://pubchem.ncbi.nlm.nih.gov | |
Description | Data deposited in or computed by PubChem | |
Molecular Weight |
194.19 g/mol | |
Source | PubChem | |
URL | https://pubchem.ncbi.nlm.nih.gov | |
Description | Data deposited in or computed by PubChem | |
Synthesis routes and methods I
Procedure details
Synthesis routes and methods II
Procedure details
Synthesis routes and methods III
Procedure details
Synthesis routes and methods IV
Procedure details
Disclaimer and Information on In-Vitro Research Products
Please be aware that all articles and product information presented on BenchChem are intended solely for informational purposes. The products available for purchase on BenchChem are specifically designed for in-vitro studies, which are conducted outside of living organisms. In-vitro studies, derived from the Latin term "in glass," involve experiments performed in controlled laboratory settings using cells or tissues. It is important to note that these products are not categorized as medicines or drugs, and they have not received approval from the FDA for the prevention, treatment, or cure of any medical condition, ailment, or disease. We must emphasize that any form of bodily introduction of these products into humans or animals is strictly prohibited by law. It is essential to adhere to these guidelines to ensure compliance with legal and ethical standards in research and experimentation.