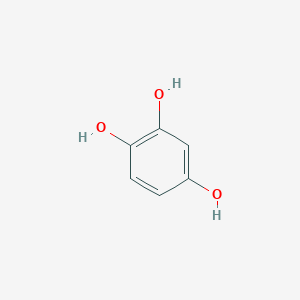
1,2,4-Benzenetriol
Overview
Description
1,2,4-Benzenetriol, also known as hydroxyhydroquinone, is an organic compound with the molecular formula C6H6O3. It is a trihydroxy derivative of benzene, where three hydroxyl groups are attached to the benzene ring at positions 1, 2, and 4. This compound is known for its role as a metabolite of benzene and its presence in roasted coffee beans .
Mechanism of Action
Target of Action
1,2,4-Benzenetriol, also known as hydroxyhydroquinone , is a phenolic metabolite of benzene . It primarily targets DNA and histones in cells . It has been shown to change DNA methylation levels at several CpG sites of erythroid-specific genes . It also affects the acetylation of histone H3 and H4 .
Mode of Action
This compound interacts with its targets by causing changes in DNA methylation and histone acetylation . This interaction results in the down-regulation of erythroid-specific genes . It has been shown to inhibit hemin-induced erythroid differentiation in K562 cells .
Biochemical Pathways
The biochemical pathways affected by this compound involve DNA methylation and histone acetylation . The compound changes DNA methylation levels at several CpG sites of erythroid-specific genes . It also affects the acetylation of histone H3 and H4 . These changes impact chromatin occupancy of GATA-1 and recruitment of RNA polymerase II at α-globin and β-globin gene clusters after hemin induction .
Pharmacokinetics
It is known to be soluble in water and polar solvents, and slightly soluble in chloroform and carbon disulfide . This suggests that it may have good bioavailability due to its solubility in water.
Result of Action
The molecular and cellular effects of this compound’s action include changes in DNA methylation and histone acetylation, leading to the down-regulation of erythroid-specific genes . This results in the inhibition of hemin-induced erythroid differentiation in K562 cells . It is also mutagenic and causes cleaving of DNA single strands by the generation of reactive oxygen species .
Action Environment
Environmental factors can influence the action, efficacy, and stability of this compound. For instance, it is known to be present in roasted coffee beans . It is also a metabolite of benzene, a ubiquitous environmental air pollutant . The concentration of this compound can reach high levels in individuals exposed to benzene . The compound’s action can also be influenced by the presence of other benzene metabolites .
Biochemical Analysis
Biochemical Properties
1,2,4-Benzenetriol readily undergoes dimerization and other reactions under mild conditions . It is reactive toward deuterium exchange with D2O, which appears pronounced under acidic conditions at room temperature . This reactivity is attributed to electrophilic aromatic substitution .
Cellular Effects
This compound has been found to inhibit hemin-induced erythroid differentiation in K562 cells . It changes DNA methylation levels at several CpG sites of erythroid-specific genes, as well as the acetylation of histone H3 and H4 . It also influences chromatin occupancy of GATA-1 and recruitment of RNA polymerase II at α-globin and β-globin gene clusters after hemin induction .
Molecular Mechanism
The molecular mechanism of this compound involves its interaction with DNA and histones. It changes DNA methylation levels and histone acetylation of erythroid-specific genes . This results in down-regulation of these genes, thereby inhibiting erythroid differentiation .
Temporal Effects in Laboratory Settings
This compound readily undergoes dimerization and other reactions under mild conditions This suggests that the effects of this compound can change over time in laboratory settings
Metabolic Pathways
Preparation Methods
1,2,4-Benzenetriol can be synthesized through several methods:
Hydrolysis of 1,2,4-triacetoxybenzene: This method involves the acid-catalyzed reaction of p-benzoquinone with acetic anhydride to form 1,2,4-triacetoxybenzene, which is then hydrolyzed to produce this compound.
Catalytic degradation of cellulose: An effective green synthesis method involves the catalytic degradation of cellulose using biomass-based activated carbon as the catalyst.
Chemical Reactions Analysis
1,2,4-Benzenetriol undergoes various chemical reactions, including:
Oxidation: It can be oxidized to form quinones and other oxidation products.
Reduction: It can be reduced to form hydroquinones.
Substitution: Electrophilic aromatic substitution reactions can occur, such as deuterium exchange with D2O under acidic conditions.
Common reagents and conditions used in these reactions include acidic or basic environments, oxidizing agents like hydrogen peroxide, and reducing agents. Major products formed from these reactions include quinones, hydroquinones, and various substituted derivatives .
Scientific Research Applications
1,2,4-Benzenetriol has a wide range of applications in scientific research:
Comparison with Similar Compounds
1,2,4-Benzenetriol can be compared with other similar compounds such as:
1,3,5-Benzenetriol: Another trihydroxybenzene derivative with hydroxyl groups at positions 1, 3, and 5.
Hydroquinone: A dihydroxybenzene derivative with hydroxyl groups at positions 1 and 4.
Catechol: A dihydroxybenzene derivative with hydroxyl groups at positions 1 and 2.
This compound is unique due to its specific hydroxyl group arrangement, which influences its reactivity and applications. Its ability to undergo selective deuteration and dimerization makes it a valuable compound for producing biobased aromatic products .
Properties
IUPAC Name |
benzene-1,2,4-triol | |
---|---|---|
Source | PubChem | |
URL | https://pubchem.ncbi.nlm.nih.gov | |
Description | Data deposited in or computed by PubChem | |
InChI |
InChI=1S/C6H6O3/c7-4-1-2-5(8)6(9)3-4/h1-3,7-9H | |
Source | PubChem | |
URL | https://pubchem.ncbi.nlm.nih.gov | |
Description | Data deposited in or computed by PubChem | |
InChI Key |
GGNQRNBDZQJCCN-UHFFFAOYSA-N | |
Source | PubChem | |
URL | https://pubchem.ncbi.nlm.nih.gov | |
Description | Data deposited in or computed by PubChem | |
Canonical SMILES |
C1=CC(=C(C=C1O)O)O | |
Source | PubChem | |
URL | https://pubchem.ncbi.nlm.nih.gov | |
Description | Data deposited in or computed by PubChem | |
Molecular Formula |
C6H6O3 | |
Source | PubChem | |
URL | https://pubchem.ncbi.nlm.nih.gov | |
Description | Data deposited in or computed by PubChem | |
DSSTOX Substance ID |
DTXSID3040930 | |
Record name | 1,2,4-Benzenetriol | |
Source | EPA DSSTox | |
URL | https://comptox.epa.gov/dashboard/DTXSID3040930 | |
Description | DSSTox provides a high quality public chemistry resource for supporting improved predictive toxicology. | |
Molecular Weight |
126.11 g/mol | |
Source | PubChem | |
URL | https://pubchem.ncbi.nlm.nih.gov | |
Description | Data deposited in or computed by PubChem | |
CAS No. |
533-73-3 | |
Record name | 1,2,4-Benzenetriol | |
Source | CAS Common Chemistry | |
URL | https://commonchemistry.cas.org/detail?cas_rn=533-73-3 | |
Description | CAS Common Chemistry is an open community resource for accessing chemical information. Nearly 500,000 chemical substances from CAS REGISTRY cover areas of community interest, including common and frequently regulated chemicals, and those relevant to high school and undergraduate chemistry classes. This chemical information, curated by our expert scientists, is provided in alignment with our mission as a division of the American Chemical Society. | |
Explanation | The data from CAS Common Chemistry is provided under a CC-BY-NC 4.0 license, unless otherwise stated. | |
Record name | 1,2,4-Benzenetriol | |
Source | ChemIDplus | |
URL | https://pubchem.ncbi.nlm.nih.gov/substance/?source=chemidplus&sourceid=0000533733 | |
Description | ChemIDplus is a free, web search system that provides access to the structure and nomenclature authority files used for the identification of chemical substances cited in National Library of Medicine (NLM) databases, including the TOXNET system. | |
Record name | Hydroxyquinol | |
Source | DTP/NCI | |
URL | https://dtp.cancer.gov/dtpstandard/servlet/dwindex?searchtype=NSC&outputformat=html&searchlist=2818 | |
Description | The NCI Development Therapeutics Program (DTP) provides services and resources to the academic and private-sector research communities worldwide to facilitate the discovery and development of new cancer therapeutic agents. | |
Explanation | Unless otherwise indicated, all text within NCI products is free of copyright and may be reused without our permission. Credit the National Cancer Institute as the source. | |
Record name | 1,2,4-Benzenetriol | |
Source | EPA Chemicals under the TSCA | |
URL | https://www.epa.gov/chemicals-under-tsca | |
Description | EPA Chemicals under the Toxic Substances Control Act (TSCA) collection contains information on chemicals and their regulations under TSCA, including non-confidential content from the TSCA Chemical Substance Inventory and Chemical Data Reporting. | |
Record name | 1,2,4-Benzenetriol | |
Source | EPA DSSTox | |
URL | https://comptox.epa.gov/dashboard/DTXSID3040930 | |
Description | DSSTox provides a high quality public chemistry resource for supporting improved predictive toxicology. | |
Record name | Benzene-1,2,4-triol | |
Source | European Chemicals Agency (ECHA) | |
URL | https://echa.europa.eu/substance-information/-/substanceinfo/100.007.797 | |
Description | The European Chemicals Agency (ECHA) is an agency of the European Union which is the driving force among regulatory authorities in implementing the EU's groundbreaking chemicals legislation for the benefit of human health and the environment as well as for innovation and competitiveness. | |
Explanation | Use of the information, documents and data from the ECHA website is subject to the terms and conditions of this Legal Notice, and subject to other binding limitations provided for under applicable law, the information, documents and data made available on the ECHA website may be reproduced, distributed and/or used, totally or in part, for non-commercial purposes provided that ECHA is acknowledged as the source: "Source: European Chemicals Agency, http://echa.europa.eu/". Such acknowledgement must be included in each copy of the material. ECHA permits and encourages organisations and individuals to create links to the ECHA website under the following cumulative conditions: Links can only be made to webpages that provide a link to the Legal Notice page. | |
Record name | 1,2,4-TRIHYDROXYBENZENE | |
Source | FDA Global Substance Registration System (GSRS) | |
URL | https://gsrs.ncats.nih.gov/ginas/app/beta/substances/173O8B04RD | |
Description | The FDA Global Substance Registration System (GSRS) enables the efficient and accurate exchange of information on what substances are in regulated products. Instead of relying on names, which vary across regulatory domains, countries, and regions, the GSRS knowledge base makes it possible for substances to be defined by standardized, scientific descriptions. | |
Explanation | Unless otherwise noted, the contents of the FDA website (www.fda.gov), both text and graphics, are not copyrighted. They are in the public domain and may be republished, reprinted and otherwise used freely by anyone without the need to obtain permission from FDA. Credit to the U.S. Food and Drug Administration as the source is appreciated but not required. | |
Synthesis routes and methods
Procedure details
Retrosynthesis Analysis
AI-Powered Synthesis Planning: Our tool employs the Template_relevance Pistachio, Template_relevance Bkms_metabolic, Template_relevance Pistachio_ringbreaker, Template_relevance Reaxys, Template_relevance Reaxys_biocatalysis model, leveraging a vast database of chemical reactions to predict feasible synthetic routes.
One-Step Synthesis Focus: Specifically designed for one-step synthesis, it provides concise and direct routes for your target compounds, streamlining the synthesis process.
Accurate Predictions: Utilizing the extensive PISTACHIO, BKMS_METABOLIC, PISTACHIO_RINGBREAKER, REAXYS, REAXYS_BIOCATALYSIS database, our tool offers high-accuracy predictions, reflecting the latest in chemical research and data.
Strategy Settings
Precursor scoring | Relevance Heuristic |
---|---|
Min. plausibility | 0.01 |
Model | Template_relevance |
Template Set | Pistachio/Bkms_metabolic/Pistachio_ringbreaker/Reaxys/Reaxys_biocatalysis |
Top-N result to add to graph | 6 |
Feasible Synthetic Routes
Disclaimer and Information on In-Vitro Research Products
Please be aware that all articles and product information presented on BenchChem are intended solely for informational purposes. The products available for purchase on BenchChem are specifically designed for in-vitro studies, which are conducted outside of living organisms. In-vitro studies, derived from the Latin term "in glass," involve experiments performed in controlled laboratory settings using cells or tissues. It is important to note that these products are not categorized as medicines or drugs, and they have not received approval from the FDA for the prevention, treatment, or cure of any medical condition, ailment, or disease. We must emphasize that any form of bodily introduction of these products into humans or animals is strictly prohibited by law. It is essential to adhere to these guidelines to ensure compliance with legal and ethical standards in research and experimentation.