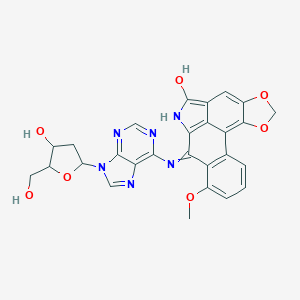
dA-Aai
Overview
Description
The compound dA-Aai is a DNA adduct formed by the interaction of aristolochic acid I (AAI) with deoxyadenosine (dA). Aristolochic acid I is a plant alkaloid known for its nephrotoxic and carcinogenic properties. The formation of this compound is significant as it is a biomarker for exposure to aristolochic acid and is associated with the development of upper urothelial cancer .
Preparation Methods
The preparation of dA-Aai involves the enzymatic activation of aristolochic acid I to reactive intermediates that can bind to DNA. This process typically occurs in vivo, where enzymes such as cytochrome P450 (CYP) and NAD(P)H:quinone oxidoreductase (NQO1) play crucial roles . The synthetic preparation of this compound in a laboratory setting involves incubating aristolochic acid I with deoxyadenosine under controlled conditions to facilitate the formation of the DNA adduct .
Chemical Reactions Analysis
dA-Aai undergoes several types of chemical reactions, including:
Oxidation: Enzymatic oxidation of aristolochic acid I to form reactive intermediates.
Reduction: Reduction of aristolochic acid I by enzymes such as NQO1 to form cyclic acylnitrenium ions.
Substitution: Covalent binding of the reactive intermediates to the exocyclic amino groups of adenine in DNA.
Common reagents and conditions used in these reactions include NADPH, cytochrome P450 enzymes, and NQO1 . The major product formed from these reactions is the this compound DNA adduct .
Scientific Research Applications
dA-Aai has several scientific research applications, including:
Biomarker for Exposure: Used as a biomarker to assess exposure to aristolochic acid in epidemiological studies.
Cancer Research: Studied for its role in the initiation and progression of upper urothelial cancer.
Pharmacology: Investigated for its potential interactions with various enzymes and its impact on drug metabolism.
Mechanism of Action
The mechanism of action of dA-Aai involves the enzymatic activation of aristolochic acid I to reactive intermediates that can bind to DNA. These intermediates form covalent bonds with the exocyclic amino groups of adenine, resulting in the formation of this compound . This DNA adduct can cause mutations, specifically A:T to T:A transversions, which are associated with the development of upper urothelial cancer . The molecular targets involved in this process include cytochrome P450 enzymes and NQO1 .
Comparison with Similar Compounds
dA-Aai is similar to other DNA adducts formed by aristolochic acid, such as dG-Aai (formed with deoxyguanosine) and this compound (formed with deoxyadenosine) . this compound is unique in its specific mutational signature and its strong association with upper urothelial cancer . Other similar compounds include:
dG-Aai: DNA adduct formed with deoxyguanosine.
These compounds share similar mechanisms of formation and biological effects but differ in their specific interactions with DNA and their mutagenic potential .
Biological Activity
Introduction
dA-Aai, or 7-deoxyadenosin-N-6-yl aristolochic acid I (this compound), is a DNA adduct formed from the metabolism of aristolochic acid I (AAI), a naturally occurring compound known for its carcinogenic properties. The biological activity of this compound is significant due to its implications in DNA damage and subsequent carcinogenesis, particularly in urothelial tumors associated with aristolochic acid nephropathy (AAN). This article reviews the biological activity of this compound, focusing on its formation, mechanisms of toxicity, and associated health risks.
Metabolic Pathways
Aristolochic acids undergo various metabolic transformations in the body, primarily in the liver and kidneys. AAI is bioactivated by enzymes such as cytochrome P450 (CYP) and NAD(P)H:quinone oxidoreductase 1 (NQO1), leading to the formation of reactive intermediates that can bind to DNA, forming adducts like this compound. The key pathways involved in the biotransformation of AAI are summarized below:
Enzyme | Role | Effect on AAI |
---|---|---|
CYP1A1 | Oxidative activation | Converts AAI to reactive forms |
NQO1 | Reductive activation | Enhances formation of DNA adducts |
CYP2C11 | Detoxification | Reduces levels of active metabolites |
Case Study Insights
Research has demonstrated that co-exposure to AAI and other aristolochic acids significantly increases the levels of this compound in vivo. For example, a study involving Wistar rats showed that treatment with an AAI/AAII mixture resulted in up to 4.5-fold higher levels of this compound compared to those treated with AAI alone. This increase correlates with enhanced carcinogenic potential due to the formation of pre-mutagenic lesions, which can lead to characteristic mutations in urothelial tumors .
Genotoxic Effects
The biological activity of this compound is primarily linked to its genotoxicity. The formation of DNA adducts like this compound can disrupt normal cellular processes, leading to mutations. These mutations are implicated in the development of various cancers, particularly bladder cancer associated with aristolochic acid exposure.
- Mechanism of Action : this compound induces mutations through transversion mutations (A:T→T:A), which are characteristic of AAI exposure. This mutagenic potential has been observed in various studies using human bladder cell lines .
Toxicological Studies
In toxicological assessments, it was found that this compound levels correlate significantly with induced mutations in specific genes such as H-ras. Elevated levels of this compound were statistically significant in studies measuring mutation frequencies (MFs) in animal models exposed to AAI .
Table: Summary of Toxicological Findings
Study Type | Findings |
---|---|
In vivo studies | Increased this compound adducts correlate with tumors |
Cell line assays | Transversion mutations observed |
Enzyme activity assays | Altered enzyme levels affecting detoxification |
Health Implications
The health implications associated with this compound are profound, particularly concerning its role in AAN and related cancers. Long-term exposure to aristolochic acids has been linked to chronic kidney disease and urothelial carcinoma. Understanding the biological activity of this compound is crucial for assessing risk factors associated with dietary intake or herbal remedies containing aristolochic acids.
Properties
IUPAC Name |
12-[9-[4-hydroxy-5-(hydroxymethyl)oxolan-2-yl]purin-6-yl]imino-14-methoxy-3,5-dioxa-10-azapentacyclo[9.7.1.02,6.08,19.013,18]nonadeca-1,6,8,11(19),13(18),14,16-heptaen-9-ol | |
---|---|---|
Source | PubChem | |
URL | https://pubchem.ncbi.nlm.nih.gov | |
Description | Data deposited in or computed by PubChem | |
InChI |
InChI=1S/C27H22N6O7/c1-37-14-4-2-3-11-18(14)21(22-19-12(27(36)32-22)5-15-24(20(11)19)39-10-38-15)31-25-23-26(29-8-28-25)33(9-30-23)17-6-13(35)16(7-34)40-17/h2-5,8-9,13,16-17,32,34-36H,6-7,10H2,1H3 | |
Source | PubChem | |
URL | https://pubchem.ncbi.nlm.nih.gov | |
Description | Data deposited in or computed by PubChem | |
InChI Key |
UJUDOVXXLPFCGM-UHFFFAOYSA-N | |
Source | PubChem | |
URL | https://pubchem.ncbi.nlm.nih.gov | |
Description | Data deposited in or computed by PubChem | |
Canonical SMILES |
COC1=CC=CC2=C1C(=NC3=C4C(=NC=N3)N(C=N4)C5CC(C(O5)CO)O)C6=C7C2=C8C(=CC7=C(N6)O)OCO8 | |
Source | PubChem | |
URL | https://pubchem.ncbi.nlm.nih.gov | |
Description | Data deposited in or computed by PubChem | |
Molecular Formula |
C27H22N6O7 | |
Source | PubChem | |
URL | https://pubchem.ncbi.nlm.nih.gov | |
Description | Data deposited in or computed by PubChem | |
Molecular Weight |
542.5 g/mol | |
Source | PubChem | |
URL | https://pubchem.ncbi.nlm.nih.gov | |
Description | Data deposited in or computed by PubChem | |
CAS No. |
127191-86-0 | |
Record name | 7-(Deoxyadenosin-N(6)-yl)aristolactam I | |
Source | ChemIDplus | |
URL | https://pubchem.ncbi.nlm.nih.gov/substance/?source=chemidplus&sourceid=0127191860 | |
Description | ChemIDplus is a free, web search system that provides access to the structure and nomenclature authority files used for the identification of chemical substances cited in National Library of Medicine (NLM) databases, including the TOXNET system. | |
Retrosynthesis Analysis
AI-Powered Synthesis Planning: Our tool employs the Template_relevance Pistachio, Template_relevance Bkms_metabolic, Template_relevance Pistachio_ringbreaker, Template_relevance Reaxys, Template_relevance Reaxys_biocatalysis model, leveraging a vast database of chemical reactions to predict feasible synthetic routes.
One-Step Synthesis Focus: Specifically designed for one-step synthesis, it provides concise and direct routes for your target compounds, streamlining the synthesis process.
Accurate Predictions: Utilizing the extensive PISTACHIO, BKMS_METABOLIC, PISTACHIO_RINGBREAKER, REAXYS, REAXYS_BIOCATALYSIS database, our tool offers high-accuracy predictions, reflecting the latest in chemical research and data.
Strategy Settings
Precursor scoring | Relevance Heuristic |
---|---|
Min. plausibility | 0.01 |
Model | Template_relevance |
Template Set | Pistachio/Bkms_metabolic/Pistachio_ringbreaker/Reaxys/Reaxys_biocatalysis |
Top-N result to add to graph | 6 |
Feasible Synthetic Routes
Disclaimer and Information on In-Vitro Research Products
Please be aware that all articles and product information presented on BenchChem are intended solely for informational purposes. The products available for purchase on BenchChem are specifically designed for in-vitro studies, which are conducted outside of living organisms. In-vitro studies, derived from the Latin term "in glass," involve experiments performed in controlled laboratory settings using cells or tissues. It is important to note that these products are not categorized as medicines or drugs, and they have not received approval from the FDA for the prevention, treatment, or cure of any medical condition, ailment, or disease. We must emphasize that any form of bodily introduction of these products into humans or animals is strictly prohibited by law. It is essential to adhere to these guidelines to ensure compliance with legal and ethical standards in research and experimentation.