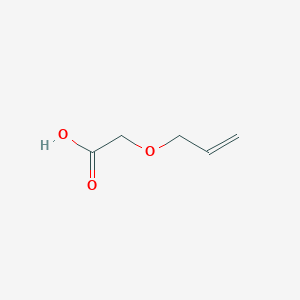
2-(Prop-2-en-1-yloxy)acetic acid
- Click on QUICK INQUIRY to receive a quote from our team of experts.
- With the quality product at a COMPETITIVE price, you can focus more on your research.
Overview
Description
2-(Prop-2-en-1-yloxy)acetic acid is an organic compound with the molecular formula C5H8O3 and a molecular weight of 116.12 g/mol . This compound is characterized by the presence of an allyloxy group attached to an acetic acid moiety.
Preparation Methods
The synthesis of 2-(Prop-2-en-1-yloxy)acetic acid typically involves the reaction of allyl alcohol with chloroacetic acid under basic conditions. The reaction proceeds via nucleophilic substitution, where the hydroxyl group of the allyl alcohol attacks the carbon atom of the chloroacetic acid, resulting in the formation of the desired product . The reaction conditions often include the use of a base such as sodium hydroxide to facilitate the nucleophilic attack and to neutralize the hydrochloric acid byproduct .
In industrial settings, the production of this compound may involve more advanced techniques and equipment to ensure high yield and purity. This can include the use of continuous flow reactors and advanced purification methods such as distillation and crystallization .
Chemical Reactions Analysis
2-(Prop-2-en-1-yloxy)acetic acid undergoes various chemical reactions, including:
Oxidation: The compound can be oxidized to form corresponding carboxylic acids or aldehydes, depending on the oxidizing agent used.
Reduction: Reduction reactions can convert the carboxylic acid group to an alcohol group.
Substitution: The allyloxy group can participate in substitution reactions, where it can be replaced by other functional groups.
Common reagents used in these reactions include oxidizing agents like potassium permanganate and reducing agents like lithium aluminum hydride . The major products formed from these reactions depend on the specific conditions and reagents used .
Scientific Research Applications
2-(Prop-2-en-1-yloxy)acetic acid has a wide range of applications in scientific research:
Chemistry: It is used as a building block in organic synthesis, particularly in the preparation of more complex molecules.
Biology: The compound can be used in studies involving enzyme inhibition and metabolic pathways.
Medicine: Research into potential therapeutic applications, including drug development and delivery systems.
Industry: It is used in the production of specialty chemicals and materials.
Mechanism of Action
The mechanism of action of 2-(Prop-2-en-1-yloxy)acetic acid involves its interaction with various molecular targets and pathways. For instance, in biological systems, it may act as an inhibitor of specific enzymes, thereby affecting metabolic processes. The allyloxy group can also participate in nucleophilic substitution reactions, which can alter the compound’s activity and interactions .
Comparison with Similar Compounds
2-(Prop-2-en-1-yloxy)acetic acid can be compared with other similar compounds such as:
- 2-Hydroxy-2-(2-hydroxy-1-naphthyl)acetic acid
- (2S)-1-Methyl-2-pyrrolidinyl)acetic acid
- (2-Amino-2-oxoethoxy)acetic acid
- (1-Methyl-2-pyrrolidinyl)acetic acid
- (2-Hydroxy-1-naphthyl)acetic acid
- Amino (2-cyclohexen-1-yl)acetic acid
- (2-Isopropylphenoxy)acetic acid
- (2-Butoxyethoxy)acetic acid
- (2-Amino-4-phenylthiazol-5-yl)acetic acid
- (2-Methoxyethoxy)acetic acid .
These compounds share structural similarities but differ in their functional groups and specific applications.
Properties
IUPAC Name |
2-prop-2-enoxyacetic acid |
Source
|
---|---|---|
Source | PubChem | |
URL | https://pubchem.ncbi.nlm.nih.gov | |
Description | Data deposited in or computed by PubChem | |
InChI |
InChI=1S/C5H8O3/c1-2-3-8-4-5(6)7/h2H,1,3-4H2,(H,6,7) |
Source
|
Source | PubChem | |
URL | https://pubchem.ncbi.nlm.nih.gov | |
Description | Data deposited in or computed by PubChem | |
InChI Key |
QXWUJRONCAPLLL-UHFFFAOYSA-N |
Source
|
Source | PubChem | |
URL | https://pubchem.ncbi.nlm.nih.gov | |
Description | Data deposited in or computed by PubChem | |
Canonical SMILES |
C=CCOCC(=O)O |
Source
|
Source | PubChem | |
URL | https://pubchem.ncbi.nlm.nih.gov | |
Description | Data deposited in or computed by PubChem | |
Molecular Formula |
C5H8O3 |
Source
|
Source | PubChem | |
URL | https://pubchem.ncbi.nlm.nih.gov | |
Description | Data deposited in or computed by PubChem | |
Molecular Weight |
116.11 g/mol |
Source
|
Source | PubChem | |
URL | https://pubchem.ncbi.nlm.nih.gov | |
Description | Data deposited in or computed by PubChem | |
CAS No. |
22577-14-6 |
Source
|
Record name | 2-(prop-2-en-1-yloxy)acetic acid | |
Source | European Chemicals Agency (ECHA) | |
URL | https://echa.europa.eu/information-on-chemicals | |
Description | The European Chemicals Agency (ECHA) is an agency of the European Union which is the driving force among regulatory authorities in implementing the EU's groundbreaking chemicals legislation for the benefit of human health and the environment as well as for innovation and competitiveness. | |
Explanation | Use of the information, documents and data from the ECHA website is subject to the terms and conditions of this Legal Notice, and subject to other binding limitations provided for under applicable law, the information, documents and data made available on the ECHA website may be reproduced, distributed and/or used, totally or in part, for non-commercial purposes provided that ECHA is acknowledged as the source: "Source: European Chemicals Agency, http://echa.europa.eu/". Such acknowledgement must be included in each copy of the material. ECHA permits and encourages organisations and individuals to create links to the ECHA website under the following cumulative conditions: Links can only be made to webpages that provide a link to the Legal Notice page. | |
Disclaimer and Information on In-Vitro Research Products
Please be aware that all articles and product information presented on BenchChem are intended solely for informational purposes. The products available for purchase on BenchChem are specifically designed for in-vitro studies, which are conducted outside of living organisms. In-vitro studies, derived from the Latin term "in glass," involve experiments performed in controlled laboratory settings using cells or tissues. It is important to note that these products are not categorized as medicines or drugs, and they have not received approval from the FDA for the prevention, treatment, or cure of any medical condition, ailment, or disease. We must emphasize that any form of bodily introduction of these products into humans or animals is strictly prohibited by law. It is essential to adhere to these guidelines to ensure compliance with legal and ethical standards in research and experimentation.