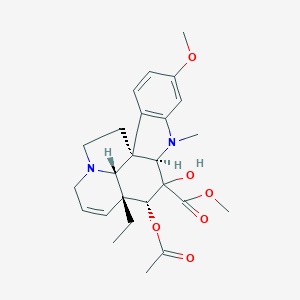
Vindoline
Overview
Description
Procaspase-activating compound 1, commonly known as PAC1, is a synthesized chemical compound that selectively induces apoptosis in cancerous cells. It was discovered in Professor Paul Hergenrother’s laboratory at the University of Illinois at Urbana–Champaign. PAC1 has gained significant attention due to its ability to activate procaspase-3, an inactive form of the enzyme caspase-3, which plays a crucial role in the execution phase of cell apoptosis .
Mechanism of Action
Target of Action
Vindoline, a vinca alkaloid, primarily targets microtubules in cells . Microtubules are crucial components of the cell’s structure and play a vital role in cell division . This compound, like other vinca alkaloids, disrupts microtubule dynamics, leading to mitotic arrest and cell death .
Mode of Action
This compound interferes with the polymerization of tubulin, a protein responsible for building the microtubule system which appears during cell division . This interference disrupts the formation of the mitotic spindle, an essential component for chromosome segregation during mitosis . As a result, the cell cycle is arrested, preventing the cell from dividing and leading to cell death .
Biochemical Pathways
The biosynthesis of this compound from tabersonine involves several steps . It starts with the hydroxylation by tabersonine 16-hydroxylase 2 (T16H2), followed by O-methylation by the 16-hydroxytabersonine O-methyltransferase (16OMT), resulting in the formation of 16-hydroxytabersonine and 16-methoxytabersonine . 16-Methoxytabersonine is then sequentially catalyzed by T3O (tabersonine 3-oxygenase; CYP71D1V2)/T3R (tabersonine 3-reductase; ALDH1), NMT (3-hydroxy-16-methoxy-2,3-dihydrotabersonine-N-methyltransferase), D4H (desacetoxythis compound-4-hydroxylase), and DAT (deacetylthis compound-4-O-acetyltransferase), leading to the biosynthesis of this compound .
Pharmacokinetics
While specific pharmacokinetic data for this compound is limited, vinca alkaloids, in general, are known to have complex pharmacokinetics . They are typically administered intravenously due to poor oral bioavailability . Metabolism of vinca alkaloids occurs primarily in the liver, mediated by cytochrome P450 enzymes . The elimination half-life, bioavailability, and other ADME properties of this compound specifically would need further investigation.
Result of Action
This compound has been shown to have various effects at the molecular and cellular level. For instance, it has been reported to reduce hyperlipidemia and renal pathophysiology in experimental type 2 diabetes . It improved the ferric reducing antioxidant power in diabetic hearts, while superoxide dismutase (SOD) oxygen radical absorbance capacity was increased in the kidneys . Furthermore, this compound restored the structure of the renal parenchyma and was accompanied by a significant decrease in the expression of caspase 9 in diabetic rats when compared to the diabetic controls .
Action Environment
The action of this compound can be influenced by various environmental factors. For instance, biotic factors like bacterial endophytes, phytoplasma, viruses, and abiotic factors such as temperature, salinity, and plant hormones like salicylic acid (SA) and methyl jasmonate (MeJa) can affect this compound accumulation in Catharanthus roseus . These factors can influence the expression of genes involved in this compound biosynthesis, thereby affecting its production .
Biochemical Analysis
Biochemical Properties
Vindoline interacts with several enzymes and proteins in its biochemical reactions. The terminal steps in the biosynthesis of this compound are catalyzed by separate acetyl coenzyme A-dependent O-acetyltransferases in Madagascar periwinkle . Two genes were isolated that had 63% nucleic acid identity and whose deduced amino acid sequences were 78% identical . These enzymes are named minovincinine-19-O-acetyltransferase (MAT) and deacetylthis compound-4-O-acetyltransferase (DAT) because they catalyze the 19-O-acetylation of indole alkaloids such as minovincinine and hörammericine and the 4-O-acetylation of deacetylthis compound, respectively .
Cellular Effects
The effects of this compound on various types of cells and cellular processes are significant. The coincident expression of tryptophan decarboxylase, strictosidine synthase, and MAT within root cortical cells suggests that the entire pathway for the biosynthesis of tabersonine and its substituted analogs occurs within these cells . This indicates that this compound influences cell function, including any impact on cell signaling pathways, gene expression, and cellular metabolism.
Molecular Mechanism
This compound exerts its effects at the molecular level through binding interactions with biomolecules, enzyme inhibition or activation, and changes in gene expression. The biosynthesis of this compound from tabersonine starts from the hydroxylation by the tabersonine 16-hydroxylase 2 (T16H2), followed by O-methylation by the 16-hydroxytabersonine O-methyltransferase (16OMT), resulting in the formation of 16-hydroxytabersonine and 16-methoxytabersonine, respectively .
Temporal Effects in Laboratory Settings
The changes in the effects of this compound over time in laboratory settings include information on the product’s stability, degradation, and any long-term effects on cellular function observed in in vitro or in vivo studies. Assays were carried out as described in experimental procedures, containing either 0.17 μg of rDAT or 6.0 μg of rMAT, respectively, along with 20 μM deacetylthis compound .
Preparation Methods
Synthetic Routes and Reaction Conditions
The synthesis of procaspase-activating compound 1 involves several steps. The key intermediate is 2-(4-benzylpiperazin-1-yl)-N-[(2-hydroxy-3-prop-2-enyl-phenyl)methylideneamino]acetamide. The synthetic route typically involves the following steps:
Formation of the Piperazine Derivative: The initial step involves the reaction of piperazine with benzyl chloride to form 4-benzylpiperazine.
Formation of the Acetamide Derivative: The next step involves the reaction of 4-benzylpiperazine with chloroacetyl chloride to form 2-(4-benzylpiperazin-1-yl)acetamide.
Formation of the Final Compound: The final step involves the reaction of 2-(4-benzylpiperazin-1-yl)acetamide with 2-hydroxy-3-prop-2-enyl-benzaldehyde under basic conditions to form procaspase-activating compound 1.
Industrial Production Methods
Industrial production of procaspase-activating compound 1 follows similar synthetic routes but on a larger scale. The process involves optimizing reaction conditions to ensure high yield and purity. The use of continuous flow reactors and automated systems can enhance the efficiency and scalability of the production process.
Chemical Reactions Analysis
Types of Reactions
Procaspase-activating compound 1 undergoes several types of chemical reactions, including:
Oxidation: The compound can undergo oxidation reactions, particularly at the phenolic hydroxyl group.
Reduction: Reduction reactions can occur at the imine group, converting it to an amine.
Substitution: The compound can undergo nucleophilic substitution reactions, particularly at the benzyl group.
Common Reagents and Conditions
Oxidation: Common oxidizing agents include hydrogen peroxide and potassium permanganate.
Reduction: Common reducing agents include sodium borohydride and lithium aluminum hydride.
Substitution: Common nucleophiles include amines and thiols.
Major Products Formed
Oxidation: The major product formed is the corresponding quinone derivative.
Reduction: The major product formed is the corresponding amine derivative.
Substitution: The major products formed are the substituted benzyl derivatives.
Scientific Research Applications
Procaspase-activating compound 1 has a wide range of scientific research applications, including:
Chemistry: It is used as a research tool to study the mechanisms of apoptosis and the role of caspases in cell death.
Biology: It is used to investigate the cellular pathways involved in apoptosis and to identify potential therapeutic targets for cancer treatment.
Medicine: It has shown potential as an anti-cancer agent and is currently being evaluated in clinical trials for the treatment of various cancers, including glioblastoma and neuroendocrine tumors
Industry: It is used in the development of new therapeutic agents and in the study of drug resistance mechanisms in cancer cells.
Comparison with Similar Compounds
Procaspase-activating compound 1 is unique in its ability to selectively activate procaspase-3 and induce apoptosis in cancerous cells. Similar compounds include:
B-PAC-1: A derivative of procaspase-activating compound 1 with enhanced potency and selectivity for procaspase-3.
TRAIL: Tumor necrosis factor-related apoptosis-inducing ligand, which induces apoptosis by binding to death receptors on the cell surface.
Caspase Activators: Other small-molecule compounds that activate caspases and induce apoptosis, such as PAC-2 and PAC-3
Procaspase-activating compound 1 stands out due to its direct activation of procaspase-3 and its potential for use in combination therapies to enhance the efficacy of other anti-cancer agents.
Properties
CAS No. |
2182-14-1 |
---|---|
Molecular Formula |
C25H32N2O6 |
Molecular Weight |
456.5 g/mol |
IUPAC Name |
methyl (1S,10R,12S)-11-acetyloxy-12-ethyl-10-hydroxy-5-methoxy-8-methyl-8,16-diazapentacyclo[10.6.1.01,9.02,7.016,19]nonadeca-2(7),3,5,13-tetraene-10-carboxylate |
InChI |
InChI=1S/C25H32N2O6/c1-6-23-10-7-12-27-13-11-24(19(23)27)17-9-8-16(31-4)14-18(17)26(3)20(24)25(30,22(29)32-5)21(23)33-15(2)28/h7-10,14,19-21,30H,6,11-13H2,1-5H3/t19?,20?,21?,23-,24-,25+/m0/s1 |
InChI Key |
CXBGOBGJHGGWIE-KVNPHLGMSA-N |
SMILES |
CCC12C=CCN3C1C4(CC3)C(C(C2OC(=O)C)(C(=O)OC)O)N(C5=C4C=CC(=C5)OC)C |
Isomeric SMILES |
CC[C@]12C=CCN3C1[C@@]4(CC3)C([C@@](C2OC(=O)C)(C(=O)OC)O)N(C5=C4C=CC(=C5)OC)C |
Canonical SMILES |
CCC12C=CCN3C1C4(CC3)C(C(C2OC(=O)C)(C(=O)OC)O)N(C5=C4C=CC(=C5)OC)C |
Appearance |
Powder |
Key on ui other cas no. |
2182-14-1 |
Pictograms |
Health Hazard |
Synonyms |
(2β,3β,4β,5α,12β,19α)-4-(Acetyloxy)-6,7-didehydro-3-hydroxy-16-methoxy-1-methyl-aspidospermidine-3-carboxylic Acid Methyl Ester; (-)-Vindoline; NSC 91994; Vindolin; |
Origin of Product |
United States |
Retrosynthesis Analysis
AI-Powered Synthesis Planning: Our tool employs the Template_relevance Pistachio, Template_relevance Bkms_metabolic, Template_relevance Pistachio_ringbreaker, Template_relevance Reaxys, Template_relevance Reaxys_biocatalysis model, leveraging a vast database of chemical reactions to predict feasible synthetic routes.
One-Step Synthesis Focus: Specifically designed for one-step synthesis, it provides concise and direct routes for your target compounds, streamlining the synthesis process.
Accurate Predictions: Utilizing the extensive PISTACHIO, BKMS_METABOLIC, PISTACHIO_RINGBREAKER, REAXYS, REAXYS_BIOCATALYSIS database, our tool offers high-accuracy predictions, reflecting the latest in chemical research and data.
Strategy Settings
Precursor scoring | Relevance Heuristic |
---|---|
Min. plausibility | 0.01 |
Model | Template_relevance |
Template Set | Pistachio/Bkms_metabolic/Pistachio_ringbreaker/Reaxys/Reaxys_biocatalysis |
Top-N result to add to graph | 6 |
Feasible Synthetic Routes
Q1: Where is vindoline primarily synthesized in the Catharanthus roseus plant?
A1: this compound biosynthesis is compartmentalized within the Catharanthus roseus plant. Early steps in the pathway, including the synthesis of the precursor 16-methoxytabersonine, occur predominantly in leaf epidermal cells. [] This precursor is then transported to other cell types within the leaf, such as mesophyll, idioblast, and laticifer cells, where the final enzymatic transformations to this compound take place. []
Q2: What is the role of light in this compound biosynthesis?
A2: Light plays a crucial role in regulating this compound biosynthesis. Studies have shown that light exposure induces the activity of key enzymes involved in the this compound pathway, such as deacetylthis compound 4-O-acetyltransferase (DAT). [, ] Phytochrome, a photoreceptor, has been implicated in this light-mediated induction of DAT activity. [] Light exposure can also influence the transcription levels of genes involved in this compound biosynthesis, such as tabersonine 16-hydroxylase (T16H) and deacetoxythis compound 4-hydroxylase (D4H). [, , ]
Q3: Does polyploidy affect this compound accumulation in Catharanthus roseus?
A3: Research suggests that polyploidy can influence this compound accumulation in Catharanthus roseus. A study found that a callus line induced from a tetraploid leaf accumulated higher levels of this compound compared to diploid callus cultures. [] This suggests that polyploidy may enhance this compound biosynthesis, potentially by influencing chlorophyll content or chloroplast development. []
Q4: What is the key enzymatic step initiating this compound biosynthesis?
A5: The hydroxylation of tabersonine at the C-16 position, catalyzed by tabersonine 16-hydroxylase (T16H), is the crucial first step in the seven-step pathway leading to this compound from tabersonine. [] Interestingly, two distinct cytochrome P450 isoforms, CYP71D12 (T16H1) and CYP71D351 (T16H2), have been identified to possess T16H activity. []
Q5: How is the expression of T16H isoforms regulated?
A6: The two T16H isoforms exhibit distinct expression patterns. T16H1 expression is limited to flowers and undifferentiated cells, whereas T16H2 expression aligns with other this compound biosynthetic genes, peaking in young leaves. [] This suggests organ-dependent regulation of tabersonine 16-hydroxylation. []
Q6: What is the role of tabersonine 3-oxygenase (T3O) and tabersonine 3-reductase (T3R) in this compound biosynthesis?
A7: T3O and T3R work in concert to form 3-hydroxy-16-methoxy-2,3-dihydrotabersonine, a key intermediate in this compound biosynthesis from tabersonine. [] T3O, a cytochrome P450 enzyme, catalyzes the initial oxidation step, while T3R, an alcohol dehydrogenase, catalyzes the subsequent reduction. [] Importantly, these enzymes seem to function in a tightly coupled manner, as the T3O reaction product is an epoxide that cannot be utilized by T3R alone. []
Disclaimer and Information on In-Vitro Research Products
Please be aware that all articles and product information presented on BenchChem are intended solely for informational purposes. The products available for purchase on BenchChem are specifically designed for in-vitro studies, which are conducted outside of living organisms. In-vitro studies, derived from the Latin term "in glass," involve experiments performed in controlled laboratory settings using cells or tissues. It is important to note that these products are not categorized as medicines or drugs, and they have not received approval from the FDA for the prevention, treatment, or cure of any medical condition, ailment, or disease. We must emphasize that any form of bodily introduction of these products into humans or animals is strictly prohibited by law. It is essential to adhere to these guidelines to ensure compliance with legal and ethical standards in research and experimentation.