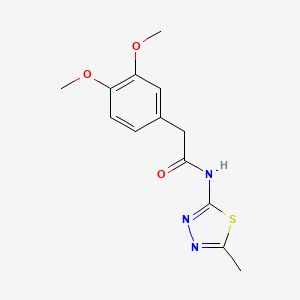
2-(3,4-dimethoxyphenyl)-N-(5-methyl-1,3,4-thiadiazol-2-yl)acetamide
- Click on QUICK INQUIRY to receive a quote from our team of experts.
- With the quality product at a COMPETITIVE price, you can focus more on your research.
Overview
Description
2-(3,4-Dimethoxyphenyl)-N-(5-methyl-1,3,4-thiadiazol-2-yl)acetamide is an organic compound that belongs to the class of acetamides This compound is characterized by the presence of a dimethoxyphenyl group and a thiadiazolyl group attached to an acetamide backbone
Preparation Methods
Synthetic Routes and Reaction Conditions
The synthesis of 2-(3,4-dimethoxyphenyl)-N-(5-methyl-1,3,4-thiadiazol-2-yl)acetamide typically involves the reaction of 3,4-dimethoxybenzaldehyde with thiosemicarbazide to form the corresponding thiosemicarbazone. This intermediate is then cyclized to form the 1,3,4-thiadiazole ring. The final step involves the acylation of the thiadiazole derivative with chloroacetyl chloride to yield the target compound.
Industrial Production Methods
While specific industrial production methods for this compound are not well-documented, the general approach would involve scaling up the laboratory synthesis procedures. This would include optimizing reaction conditions such as temperature, solvent, and catalysts to ensure high yield and purity of the final product.
Chemical Reactions Analysis
Types of Reactions
2-(3,4-Dimethoxyphenyl)-N-(5-methyl-1,3,4-thiadiazol-2-yl)acetamide can undergo various chemical reactions, including:
Oxidation: The compound can be oxidized to form corresponding sulfoxides or sulfones.
Reduction: Reduction reactions can convert the thiadiazole ring to a thioether or other reduced forms.
Substitution: The aromatic ring can undergo electrophilic substitution reactions, such as nitration or halogenation.
Common Reagents and Conditions
Oxidation: Common oxidizing agents include hydrogen peroxide and m-chloroperbenzoic acid.
Reduction: Reducing agents such as lithium aluminum hydride or sodium borohydride are typically used.
Substitution: Electrophilic substitution reactions often use reagents like nitric acid for nitration or bromine for bromination.
Major Products Formed
Oxidation: Sulfoxides and sulfones.
Reduction: Thioethers and other reduced derivatives.
Substitution: Nitro or halogenated derivatives of the aromatic ring.
Scientific Research Applications
2-(3,4-Dimethoxyphenyl)-N-(5-methyl-1,3,4-thiadiazol-2-yl)acetamide has several applications in scientific research:
Chemistry: Used as a building block for the synthesis of more complex molecules.
Biology: Investigated for its potential biological activities, including antimicrobial and anticancer properties.
Medicine: Explored as a potential therapeutic agent due to its unique chemical structure.
Industry: Utilized in the development of new materials and chemical processes.
Mechanism of Action
The mechanism of action of 2-(3,4-dimethoxyphenyl)-N-(5-methyl-1,3,4-thiadiazol-2-yl)acetamide involves its interaction with specific molecular targets. The compound may inhibit certain enzymes or receptors, leading to its observed biological effects. The exact pathways and targets can vary depending on the specific application and context of use.
Comparison with Similar Compounds
Similar Compounds
2-(3,4-Dimethoxyphenyl)-N-(1,3,4-thiadiazol-2-yl)acetamide: Lacks the methyl group on the thiadiazole ring.
2-(3,4-Dimethoxyphenyl)-N-(5-methyl-1,3,4-thiadiazol-2-yl)propionamide: Has a propionamide group instead of an acetamide group.
Uniqueness
2-(3,4-Dimethoxyphenyl)-N-(5-methyl-1,3,4-thiadiazol-2-yl)acetamide is unique due to the presence of both the dimethoxyphenyl and methyl-thiadiazole groups, which confer specific chemical and biological properties not found in closely related compounds.
Properties
IUPAC Name |
2-(3,4-dimethoxyphenyl)-N-(5-methyl-1,3,4-thiadiazol-2-yl)acetamide |
Source
|
---|---|---|
Source | PubChem | |
URL | https://pubchem.ncbi.nlm.nih.gov | |
Description | Data deposited in or computed by PubChem | |
InChI |
InChI=1S/C13H15N3O3S/c1-8-15-16-13(20-8)14-12(17)7-9-4-5-10(18-2)11(6-9)19-3/h4-6H,7H2,1-3H3,(H,14,16,17) |
Source
|
Source | PubChem | |
URL | https://pubchem.ncbi.nlm.nih.gov | |
Description | Data deposited in or computed by PubChem | |
InChI Key |
FKEUHFIXZRJOJQ-UHFFFAOYSA-N |
Source
|
Source | PubChem | |
URL | https://pubchem.ncbi.nlm.nih.gov | |
Description | Data deposited in or computed by PubChem | |
Canonical SMILES |
CC1=NN=C(S1)NC(=O)CC2=CC(=C(C=C2)OC)OC |
Source
|
Source | PubChem | |
URL | https://pubchem.ncbi.nlm.nih.gov | |
Description | Data deposited in or computed by PubChem | |
Molecular Formula |
C13H15N3O3S |
Source
|
Source | PubChem | |
URL | https://pubchem.ncbi.nlm.nih.gov | |
Description | Data deposited in or computed by PubChem | |
Molecular Weight |
293.34 g/mol |
Source
|
Source | PubChem | |
URL | https://pubchem.ncbi.nlm.nih.gov | |
Description | Data deposited in or computed by PubChem | |
Disclaimer and Information on In-Vitro Research Products
Please be aware that all articles and product information presented on BenchChem are intended solely for informational purposes. The products available for purchase on BenchChem are specifically designed for in-vitro studies, which are conducted outside of living organisms. In-vitro studies, derived from the Latin term "in glass," involve experiments performed in controlled laboratory settings using cells or tissues. It is important to note that these products are not categorized as medicines or drugs, and they have not received approval from the FDA for the prevention, treatment, or cure of any medical condition, ailment, or disease. We must emphasize that any form of bodily introduction of these products into humans or animals is strictly prohibited by law. It is essential to adhere to these guidelines to ensure compliance with legal and ethical standards in research and experimentation.