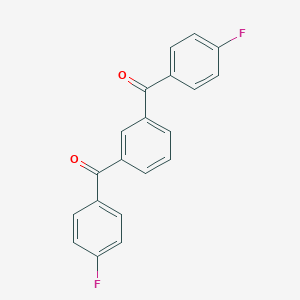
1,3-Bis(4-fluorobenzoyl)benzene
Overview
Description
1,3-Bis(4-fluorobenzoyl)benzene is an organic compound with the molecular formula C20H12F2O2. It is a fluorinated building block used in various chemical syntheses. This compound is known for its ability to polymerize with other compounds to form high molecular weight polymers, making it valuable in materials science and polymer chemistry .
Mechanism of Action
Target of Action
1,3-Bis(4-fluorobenzoyl)benzene is an organic building block
Mode of Action
The compound interacts with its targets through a process of polymerization with 4,4′-isopropylidenediphenol (bisphenol-A) in the presence of N-methyl-2-pyrrolidinone (solvent) to afford high molecular weight polymers .
Biochemical Pathways
The biochemical pathways affected by this compound involve the synthesis of sulfonated poly (aryl ether ketone)s (SPAEK) copolymers by aromatic nucleophilic polycondensation
Result of Action
The result of the action of this compound is the formation of high molecular weight polymers . It may also be employed as an activated nonsulfonated difluoro-monomer for the synthesis of fluorenyl-containing sulfonated poly (aryl ether ether ketone ketone)s .
Biochemical Analysis
Biochemical Properties
It is known to be used for the synthesis of sulfonated poly (aryl ether ketone)s (SPAEK) copolymers by aromatic nucleophilic polycondensation . This suggests that it may interact with various enzymes and proteins involved in these processes.
Molecular Mechanism
It is known to be involved in the synthesis of SPAEK copolymers , suggesting that it may have binding interactions with biomolecules, and could potentially influence enzyme activity and gene expression.
Preparation Methods
Synthetic Routes and Reaction Conditions
1,3-Bis(4-fluorobenzoyl)benzene can be synthesized through aromatic nucleophilic polycondensation. One common method involves the reaction of 4-fluorobenzoyl chloride with 1,3-dihydroxybenzene in the presence of a base such as pyridine. The reaction typically occurs under reflux conditions, and the product is purified through recrystallization .
Industrial Production Methods
In industrial settings, the production of this compound may involve large-scale aromatic nucleophilic polycondensation reactions. The use of continuous flow reactors and optimized reaction conditions can enhance yield and purity. Solvents like N-methyl-2-pyrrolidinone are often employed to facilitate the polymerization process .
Chemical Reactions Analysis
Types of Reactions
1,3-Bis(4-fluorobenzoyl)benzene undergoes various chemical reactions, including:
Substitution Reactions: The fluorine atoms can be substituted with other functional groups under appropriate conditions.
Polymerization: It can polymerize with compounds like bisphenol-A to form high molecular weight polymers
Common Reagents and Conditions
Substitution Reactions: Common reagents include nucleophiles such as amines or thiols. The reactions typically occur under mild conditions with the use of catalysts.
Polymerization: Solvents like N-methyl-2-pyrrolidinone and bases such as pyridine are commonly used
Major Products Formed
Substitution Reactions: Products include various substituted derivatives of this compound.
Polymerization: High molecular weight polymers, such as sulfonated poly(aryl ether ketone)s (SPAEK), are formed
Scientific Research Applications
1,3-Bis(4-fluorobenzoyl)benzene has several scientific research applications:
Chemistry: Used as a building block in the synthesis of advanced polymers and materials.
Biology: Employed in studies involving the uptake of fluorinated compounds in biological systems.
Industry: Utilized in the production of high-performance polymers for various industrial applications
Comparison with Similar Compounds
Similar Compounds
- 4,4′-Difluorobenzophenone
- 4-Fluorobenzophenone
- 1,4-Dibenzoylbenzene
- 4,4′-Dihydroxybenzophenone
Uniqueness
1,3-Bis(4-fluorobenzoyl)benzene is unique due to its dual fluorine substitution, which enhances its reactivity and ability to form high molecular weight polymers. This makes it particularly valuable in the synthesis of advanced materials and polymers compared to its similar compounds .
Properties
IUPAC Name |
[3-(4-fluorobenzoyl)phenyl]-(4-fluorophenyl)methanone | |
---|---|---|
Source | PubChem | |
URL | https://pubchem.ncbi.nlm.nih.gov | |
Description | Data deposited in or computed by PubChem | |
InChI |
InChI=1S/C20H12F2O2/c21-17-8-4-13(5-9-17)19(23)15-2-1-3-16(12-15)20(24)14-6-10-18(22)11-7-14/h1-12H | |
Source | PubChem | |
URL | https://pubchem.ncbi.nlm.nih.gov | |
Description | Data deposited in or computed by PubChem | |
InChI Key |
PISLKPDKKIDMQT-UHFFFAOYSA-N | |
Source | PubChem | |
URL | https://pubchem.ncbi.nlm.nih.gov | |
Description | Data deposited in or computed by PubChem | |
Canonical SMILES |
C1=CC(=CC(=C1)C(=O)C2=CC=C(C=C2)F)C(=O)C3=CC=C(C=C3)F | |
Source | PubChem | |
URL | https://pubchem.ncbi.nlm.nih.gov | |
Description | Data deposited in or computed by PubChem | |
Molecular Formula |
C20H12F2O2 | |
Source | PubChem | |
URL | https://pubchem.ncbi.nlm.nih.gov | |
Description | Data deposited in or computed by PubChem | |
DSSTOX Substance ID |
DTXSID20350852 | |
Record name | 1,3-Bis(4-fluorobenzoyl)benzene | |
Source | EPA DSSTox | |
URL | https://comptox.epa.gov/dashboard/DTXSID20350852 | |
Description | DSSTox provides a high quality public chemistry resource for supporting improved predictive toxicology. | |
Molecular Weight |
322.3 g/mol | |
Source | PubChem | |
URL | https://pubchem.ncbi.nlm.nih.gov | |
Description | Data deposited in or computed by PubChem | |
CAS No. |
108464-88-6 | |
Record name | 1,3-Bis(4-fluorobenzoyl)benzene | |
Source | EPA DSSTox | |
URL | https://comptox.epa.gov/dashboard/DTXSID20350852 | |
Description | DSSTox provides a high quality public chemistry resource for supporting improved predictive toxicology. | |
Synthesis routes and methods I
Procedure details
Synthesis routes and methods II
Procedure details
Retrosynthesis Analysis
AI-Powered Synthesis Planning: Our tool employs the Template_relevance Pistachio, Template_relevance Bkms_metabolic, Template_relevance Pistachio_ringbreaker, Template_relevance Reaxys, Template_relevance Reaxys_biocatalysis model, leveraging a vast database of chemical reactions to predict feasible synthetic routes.
One-Step Synthesis Focus: Specifically designed for one-step synthesis, it provides concise and direct routes for your target compounds, streamlining the synthesis process.
Accurate Predictions: Utilizing the extensive PISTACHIO, BKMS_METABOLIC, PISTACHIO_RINGBREAKER, REAXYS, REAXYS_BIOCATALYSIS database, our tool offers high-accuracy predictions, reflecting the latest in chemical research and data.
Strategy Settings
Precursor scoring | Relevance Heuristic |
---|---|
Min. plausibility | 0.01 |
Model | Template_relevance |
Template Set | Pistachio/Bkms_metabolic/Pistachio_ringbreaker/Reaxys/Reaxys_biocatalysis |
Top-N result to add to graph | 6 |
Feasible Synthetic Routes
ANone: 1,3-Bis(4-fluorobenzoyl)benzene has the molecular formula C20H12F2O2 and a molecular weight of 322.30 g/mol.
A: Researchers commonly use techniques like FTIR, 1H NMR, and 13C NMR to characterize this compound. [, , ] These methods provide information on the presence of functional groups, the arrangement of hydrogen and carbon atoms, and can confirm the successful synthesis of the compound.
A: this compound acts as an activated aromatic difluoride, undergoing nucleophilic aromatic substitution (SNAr) reactions with bisphenol monomers. [, , ] This reactivity is key to its use in synthesizing various poly(arylene ether ketone)s and related polymers.
A: Reaction conditions like solvent, temperature, and the choice of base can significantly influence the polymerization process. [, ] For instance, using different alkali metal carbonates can lead to variations in the polymer's sequence distribution and crystallinity. []
ANone: This monomer serves as a building block for a diverse range of polymers, including:
- Poly(arylene ether ketone)s (PAEKs) [, , ]
- Poly(ether ether ketone ketone)s (PEEKKs) [, , , ]
- Poly(phthalazinone ether ketone ketone)s [, ]
- Poly(arylene ether benzimidazole)s []
- Poly(arylene thioether ketone ketone sulfone)s []
ANone: These polymers often exhibit:
A: This monomer is soluble in organic solvents like N-methyl-2-pyrrolidinone (NMP), dimethylformamide (DMF), and dimethylacetamide (DMAc), facilitating its use in polymerization reactions. [, , ]
A: Introducing specific structural features, such as pendant groups or different co-monomers, allows for fine-tuning polymer properties like solubility, glass transition temperature, and mechanical strength. [, , ] For instance, incorporating bulky pendant groups can enhance solubility. []
A: These polymers are known for their high thermal stability, with some exhibiting 5% weight loss temperatures exceeding 500°C. [, ] This stability is crucial for applications involving high operating temperatures.
A: The presence of different linkages (ether, ketone, sulfone) and aromatic units influences the polymer's susceptibility to thermal and oxidative degradation. [] Research on structure-property relationships helps in designing polymers with improved stability.
ANone: As with any chemical, appropriate safety data sheets should be consulted. Generally, it's advisable to handle this compound using personal protective equipment in a well-ventilated area.
A: Research into the environmental impact of these materials is ongoing. [] Exploring sustainable synthesis routes, biodegradable polymer designs, and efficient recycling methods are important areas of focus. []
Disclaimer and Information on In-Vitro Research Products
Please be aware that all articles and product information presented on BenchChem are intended solely for informational purposes. The products available for purchase on BenchChem are specifically designed for in-vitro studies, which are conducted outside of living organisms. In-vitro studies, derived from the Latin term "in glass," involve experiments performed in controlled laboratory settings using cells or tissues. It is important to note that these products are not categorized as medicines or drugs, and they have not received approval from the FDA for the prevention, treatment, or cure of any medical condition, ailment, or disease. We must emphasize that any form of bodily introduction of these products into humans or animals is strictly prohibited by law. It is essential to adhere to these guidelines to ensure compliance with legal and ethical standards in research and experimentation.