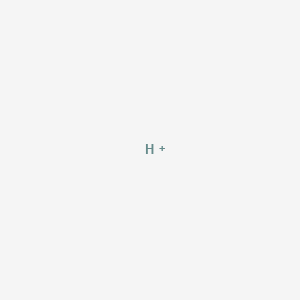
Hydron
- Click on QUICK INQUIRY to receive a quote from our team of experts.
- With the quality product at a COMPETITIVE price, you can focus more on your research.
Overview
Description
Based on the provided evidence, two primary definitions emerge:
Comparison with Similar Compounds
Hydron as a Hydrogen Cation (Chemical Term)
In chemistry, "this compound" is the IUPAC-endorsed general term for the cation of hydrogen, encompassing its isotopes:
- Protium ion (¹H⁺) : Commonly referred to as a proton.
- Deuterium ion (²H⁺ or D⁺) : A stable isotope with one neutron.
- Tritium ion (³H⁺ or T⁺) : A radioactive isotope with two neutrons .
These ions differ in mass and nuclear stability but share identical charge (+1) and chemical reactivity due to their electronic structure.
This compound as a Polyhydroxyethylmethacrylate (PHEMA) Gel (Medical Material)
In medical literature, "this compound" refers to a polyhydroxyethylmethacrylate gel used in reconstructive surgery and contact lenses. It is a biocompatible hydrogel with high water content, enabling applications in tissue engineering and drug delivery .
Comparison with Similar Compounds/Species
This compound (Hydrogen Cations) vs. Isotopic Analogues
The isotopic variants of this compound (¹H⁺, ²H⁺, ³H⁺) are compared below:
Property | Protium (¹H⁺) | Deuterium (²H⁺) | Tritium (³H⁺) |
---|---|---|---|
Natural Abundance | 99.98% | 0.02% | Trace (radioactive) |
Mass (Da) | 1.007 | 2.014 | 3.016 |
Half-Life | Stable | Stable | 12.3 years |
Applications | Acid-base chemistry | NMR spectroscopy | Radiopharmaceuticals |
Key Findings :
- Deuterium’s higher mass makes it valuable in kinetic isotope effect studies and nuclear magnetic resonance (NMR) .
- Tritium’s radioactivity limits its use to controlled environments, such as tracer experiments .
This compound (PHEMA Gel) vs. Biomedical Hydrogels
This compound (PHEMA) is compared to other hydrogels used in medical applications:
Key Findings :
- PHEMA’s moderate water content and strength make it ideal for long-term implants, while collagen hydrogels excel in biodegradability for temporary scaffolds .
- Clinical studies report minimal inflammatory response to this compound in subcutaneous implants, though long-term stability requires further validation .
Critical Analysis of Evidence
Preparation Methods
Steam Methane Reforming (SMR)
Steam methane reforming (SMR) is the most widely adopted method for industrial hydrogen production, accounting for approximately 95% of global output . This thermochemical process involves reacting methane (CH₄) with high-temperature steam (700–1,000°C) in the presence of a nickel-based catalyst to produce synthesis gas (syngas), a mixture of hydrogen (H₂), carbon monoxide (CO), and carbon dioxide (CO₂). The primary reactions are:
\ceCH4(g)+H2O(g)−>3H2(g)+CO(g)ΔH=+206kJ/mol[4]
\ceCO(g)+H2O(g)<=>H2(g)+CO2(g)ΔH=−41kJ/mol[4]
The water-gas shift reaction (second equation) enhances hydrogen yield by converting CO and steam into additional H₂ and CO₂. Modern SMR plants integrate carbon capture and storage (CCS) technologies to mitigate CO₂ emissions, reducing the carbon footprint by up to 90% . However, SMR remains energy-intensive, requiring 3.5–4.5 kWh per cubic meter of H₂ produced, and depends on fossil fuel feedstocks .
Economic and Technical Data:
Parameter | Value | Source |
---|---|---|
Capital Cost | $1,000–$1,500 per kW | |
Efficiency | 70–85% | |
CO₂ Emissions (with CCS) | 0.5–1.0 kg CO₂/kg H₂ |
Biomass Gasification
Biomass gasification offers a renewable pathway for hydrogen production by converting organic materials (e.g., agricultural waste, wood chips) into syngas through partial oxidation at 800–1,200°C . The process involves three stages:
-
Pyrolysis : Thermal decomposition of biomass into volatile gases, tar, and char.
-
Oxidation : Char reacts with oxygen to produce CO and heat.
-
Reduction : CO and steam undergo the water-gas shift reaction to yield H₂ .
The overall reaction can be simplified as:
\ceC6H10O5+6H2O−>12H2+6CO2[2]
Biomass gasification achieves 50–60% efficiency and produces 12–18 kg of H₂ per ton of dry biomass . Challenges include tar formation, which clogs reactors, and the need for extensive feedstock preprocessing. Recent advancements in fluidized-bed gasifiers and catalytic tar cracking have improved operational reliability .
Water Electrolysis
Electrolysis splits water into H₂ and O₂ using electricity, with purity levels exceeding 99.95% . Three electrolyzer technologies dominate:
Alkaline Electrolyzers
Alkaline systems employ a liquid electrolyte (e.g., potassium hydroxide) and nickel electrodes. They operate at 70–90°C with efficiencies of 60–70% . Despite their maturity, they suffer from slow response times and limited compatibility with intermittent renewable energy sources.
Proton Exchange Membrane (PEM) Electrolyzers
PEM systems use a solid polymer electrolyte and platinum catalysts, enabling rapid startup and dynamic operation. Efficiency ranges from 65–80%, with capital costs of $1,400–$2,100 per kW . Recent research at the University of Arkansas demonstrated that nickel-iron core-shell nanoparticles reduce overpotentials by 30%, lowering energy consumption .
Solid Oxide Electrolyzers (SOEC)
SOECs operate at 700–900°C, utilizing ceramic electrolytes to achieve efficiencies up to 90% . High temperatures enable thermal integration with industrial processes, but material degradation remains a barrier to commercialization.
Comparative Performance of Electrolyzers:
Type | Efficiency | Capital Cost ($/kW) | Lifetime (hours) |
---|---|---|---|
Alkaline | 60–70% | 800–1,500 | 60,000–90,000 |
PEM | 65–80% | 1,400–2,100 | 40,000–60,000 |
SOEC | 80–90% | 2,000–3,000 | 20,000–30,000 |
Data synthesized from . |
Solar Thermochemical Hydrogen (STCH)
STCH employs concentrated solar energy to drive redox cycles for water splitting. For example, metal oxides (e.g., cerium(IV) oxide) undergo reduction at 1,400–1,600°C, followed by steam re-oxidation to produce H₂ :
\ce2CeO2−>Ce2O3+0.5O2(Solar Reduction)
\ceCe2O3+H2O−>2CeO2+H2(Oxidation)
STCH achieves theoretical efficiencies of 40–50% but faces challenges in reactor design and material stability. Pilot projects at the National Renewable Energy Laboratory (NREL) have demonstrated continuous H₂ production at 3 kg/day .
Biological Hydrogen Production
Microbial fermentation and photobiological methods utilize bacteria or algae to convert biomass or sunlight into H₂. Clostridium species ferment carbohydrates into H₂, acetate, and butyrate via the dark fermentation pathway, yielding 1–2 mol H₂ per mole of glucose . Photobiological systems, such as green algae, employ hydrogenases to split water under anaerobic conditions, though efficiencies remain below 1% .
Emerging Technologies and Innovations
Photocatalytic Water Splitting
Nanostructured photocatalysts (e.g., TiO₂, CdS) use sunlight to drive water splitting. Recent breakthroughs in Z-scheme systems, which mimic natural photosynthesis, have achieved 8% solar-to-hydrogen efficiency in lab settings .
Plasma-Driven Reforming
Non-thermal plasma reactors enable methane reforming at lower temperatures (200–400°C) by generating reactive species (e.g., radicals, ions). This approach reduces coke formation and enhances H₂ selectivity to 80% .
Properties
CAS No. |
12408-02-5 |
---|---|
Molecular Formula |
H+ |
Molecular Weight |
1.008 g/mol |
IUPAC Name |
hydron |
InChI |
InChI=1S/p+1 |
InChI Key |
GPRLSGONYQIRFK-UHFFFAOYSA-N |
SMILES |
[H+] |
Canonical SMILES |
[H+] |
physical_description |
Solid |
Synonyms |
Hydrogen Ion Hydrogen Ions Ion, Hydrogen Ions, Hydrogen Proton Protons |
Origin of Product |
United States |
Disclaimer and Information on In-Vitro Research Products
Please be aware that all articles and product information presented on BenchChem are intended solely for informational purposes. The products available for purchase on BenchChem are specifically designed for in-vitro studies, which are conducted outside of living organisms. In-vitro studies, derived from the Latin term "in glass," involve experiments performed in controlled laboratory settings using cells or tissues. It is important to note that these products are not categorized as medicines or drugs, and they have not received approval from the FDA for the prevention, treatment, or cure of any medical condition, ailment, or disease. We must emphasize that any form of bodily introduction of these products into humans or animals is strictly prohibited by law. It is essential to adhere to these guidelines to ensure compliance with legal and ethical standards in research and experimentation.