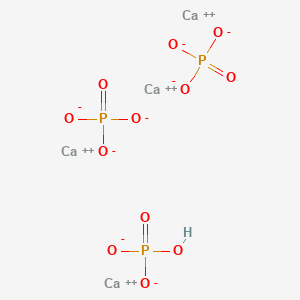
Octacalcium phosphate
Overview
Description
Octacalcium phosphate is a calcium phosphate compound with the chemical formula ( \text{Ca}_8\text{H}_2(\text{PO}_4)_6 \cdot 5\text{H}_2\text{O} ). It is recognized as a precursor to hydroxyapatite, which is a major component of bone and tooth enamel. This compound is known for its biocompatibility and osteoconductivity, making it a valuable material in biomedical applications, particularly in bone tissue engineering and regeneration.
Mechanism of Action
Target of Action
Octacalcium phosphate (OCP) is a form of calcium phosphate that plays a crucial role in biological systems . It primarily targets bone tissue and is considered a precursor to tooth enamel, dentine, and bones . OCP has unique properties among calcium phosphate compounds, as it can incorporate carboxylate ions into its layered crystal structure . This specific crystallographic property could lead to the development of various materials, including biomaterials .
Mode of Action
OCP interacts with its targets by acting as a precursor of hydroxyapatite (HA), an inorganic biomineral that is vital in bone growth . The two-layered structure of OCP, composed of an apatitic layer and a hydrated layer, is intensively involved in ion-exchange surface reactions . This results in OCP hydrolysis to HA and adsorption of ions or molecular groups present in the environment . The transformation of OCP to HA is a well-known phenomenon, although its exact mechanism remains unclear .
Biochemical Pathways
The biochemical pathways affected by OCP primarily involve the formation of apatites in enamel, dentine, and bone . In previous research, OCP was implanted into calvaria and thigh muscle of mice and found to transform into low crystalline hydroxyapatite . This transformation promotes osteoblastic cell differentiation in vitro and facilitates bone regeneration in vivo .
Pharmacokinetics
It’s known that ocp exhibits good biocompatibility and osseointegration rate compared to other calcium phosphates .
Result of Action
The result of OCP’s action is the promotion of bone growth and regeneration . OCP enhances bone regeneration and is resorbed in vivo more than HA or βTCP . It has been shown that, in the course of OCP conversion to hydroxyapatite (HAP), the crystal lattice of OCP experiences topological changes as a result of Ca2+ and PO43– diffusion .
Action Environment
The action of OCP is influenced by various environmental factors. For instance, the composition of the environmental solution affects the degree and rate of OCP hydrolysis, its surface reactivity, and further in vitro and in vivo properties . Moreover, the temperature and pH of the reaction environment can significantly affect the formation and stability of OCP . Particularly, unhydrolyzed carbamide could stabilize newly formed pure OCP crystals even at 90 °C .
Biochemical Analysis
Biochemical Properties
OCP plays a significant role in biochemical reactions. It interacts with various enzymes, proteins, and other biomolecules. The nature of these interactions is complex and multifaceted. For instance, during the conversion of OCP to hydroxyapatite, the crystal lattice of OCP undergoes topological changes as a result of Ca2+ and PO43– diffusion .
Cellular Effects
Octacalcium phosphate has profound effects on various types of cells and cellular processes. It influences cell function, including impacts on cell signaling pathways, gene expression, and cellular metabolism. For example, OCP has been shown to promote osteoblastic cell differentiation in vitro and facilitate bone regeneration in vivo .
Molecular Mechanism
The mechanism of action of this compound is complex. It exerts its effects at the molecular level, including binding interactions with biomolecules, enzyme inhibition or activation, and changes in gene expression. The process of OCP conversion to hydroxyapatite involves Ca2+ and PO43– diffusion, indicating a complex interaction with biomolecules .
Temporal Effects in Laboratory Settings
In laboratory settings, the effects of this compound change over time. Information on the product’s stability, degradation, and any long-term effects on cellular function observed in in vitro or in vivo studies is crucial. For instance, OCP loses water starting at a temperature near 90°C, and heating to 300°C causes transformations accompanied by pyrophosphate formation from OCP .
Dosage Effects in Animal Models
The effects of this compound vary with different dosages in animal models. Studies have shown that OCP enhances bone regeneration and is resorbed in vivo more than HA or βTCP
Preparation Methods
Synthetic Routes and Reaction Conditions: Octacalcium phosphate can be synthesized through various methods, including:
Direct Precipitation: This involves the dropwise addition of calcium acetate solution into a sodium acid phosphate solution, maintained at a pH of 5 or 6 and a temperature of 60°C for 3 to 4 hours.
Hydrolysis of α-Tricalcium Phosphate: This method involves the hydrolysis of α-tricalcium phosphate in the presence of water, resulting in the formation of this compound.
Micro-Flow Reactor: A micro-flow reactor can be used to mix aqueous solutions of calcium acetate and sodium phosphate monobasic, resulting in the formation of well-defined this compound particles.
Industrial Production Methods: Industrial production of this compound typically involves the use of controlled precipitation methods to ensure the purity and consistency of the product. Factors such as reaction volume, stirring rate, and flow rate are carefully managed to achieve the desired product characteristics .
Chemical Reactions Analysis
Types of Reactions: Octacalcium phosphate undergoes various chemical reactions, including:
Hydrolysis: this compound can hydrolyze to form hydroxyapatite, especially in physiological conditions.
Ion Exchange: It can participate in ion-exchange reactions, where ions such as sodium or potassium can replace calcium ions in the structure.
Common Reagents and Conditions:
Hydrolysis: Typically involves water and physiological pH conditions.
Ion Exchange: Involves the use of solutions containing the desired exchange ions, such as sodium chloride or potassium chloride.
Major Products Formed:
Hydroxyapatite: Formed through the hydrolysis of this compound.
Ion-Exchanged this compound: Formed through ion-exchange reactions.
Scientific Research Applications
Octacalcium phosphate has a wide range of scientific research applications, including:
Bone Tissue Engineering: Due to its biocompatibility and osteoconductivity, this compound is used as a scaffold material for bone regeneration.
Dental Applications: It is used in dental materials for the repair and regeneration of tooth enamel.
Biomaterials Research: this compound is studied for its potential to incorporate various ions, enhancing its properties for specific biomedical applications.
Electrochemical Devices: Research has shown its potential use in capacitive behavior as an electrode material in supercapacitors.
Comparison with Similar Compounds
Hydroxyapatite: A major component of bone and tooth enamel, with a similar structure to octacalcium phosphate but with a higher calcium-to-phosphate ratio.
Tricalcium Phosphate: Another calcium phosphate compound used in bone grafts and dental materials.
Dicalcium Phosphate: Used as a dietary supplement and in various industrial applications.
Uniqueness of this compound: this compound is unique due to its ability to act as a precursor to hydroxyapatite, its biocompatibility, and its osteoconductivity. Its layered structure allows for the incorporation of various ions, enhancing its properties for specific applications .
Properties
CAS No. |
13767-12-9 |
---|---|
Molecular Formula |
CaH3O4P |
Molecular Weight |
138.07 g/mol |
IUPAC Name |
tetracalcium;hydrogen phosphate;diphosphate |
InChI |
InChI=1S/Ca.H3O4P/c;1-5(2,3)4/h;(H3,1,2,3,4) |
InChI Key |
UUVBYOGFRMMMQL-UHFFFAOYSA-N |
SMILES |
OP(=O)([O-])[O-].[O-]P(=O)([O-])[O-].[O-]P(=O)([O-])[O-].[Ca+2].[Ca+2].[Ca+2].[Ca+2] |
Canonical SMILES |
OP(=O)(O)O.[Ca] |
13767-12-9 | |
Related CAS |
14096-86-7 (calcium-3/4H3PO4-5/8H2O) |
Synonyms |
octa-calcium phosphate octacalcium phosphate octacalcium phosphate hydrate |
Origin of Product |
United States |
Retrosynthesis Analysis
AI-Powered Synthesis Planning: Our tool employs the Template_relevance Pistachio, Template_relevance Bkms_metabolic, Template_relevance Pistachio_ringbreaker, Template_relevance Reaxys, Template_relevance Reaxys_biocatalysis model, leveraging a vast database of chemical reactions to predict feasible synthetic routes.
One-Step Synthesis Focus: Specifically designed for one-step synthesis, it provides concise and direct routes for your target compounds, streamlining the synthesis process.
Accurate Predictions: Utilizing the extensive PISTACHIO, BKMS_METABOLIC, PISTACHIO_RINGBREAKER, REAXYS, REAXYS_BIOCATALYSIS database, our tool offers high-accuracy predictions, reflecting the latest in chemical research and data.
Strategy Settings
Precursor scoring | Relevance Heuristic |
---|---|
Min. plausibility | 0.01 |
Model | Template_relevance |
Template Set | Pistachio/Bkms_metabolic/Pistachio_ringbreaker/Reaxys/Reaxys_biocatalysis |
Top-N result to add to graph | 6 |
Feasible Synthetic Routes
Q1: What is the molecular formula and weight of octacalcium phosphate?
A1: The molecular formula of this compound is Ca8H2(PO4)6·5H2O [, ]. Its molecular weight is 1004.64 g/mol.
Q2: Which spectroscopic techniques are commonly used to characterize this compound?
A2: Common spectroscopic techniques used to characterize OCP include X-ray diffraction (XRD), Fourier-transform infrared spectroscopy (FTIR), solid-state 31P nuclear magnetic resonance (NMR), and Raman spectroscopy [, , , , ].
Q3: What are the main structural differences between this compound and hydroxyapatite?
A3: Both OCP and hydroxyapatite (HA) are calcium phosphate compounds, but OCP is a precursor to HA in biological mineralization. OCP has a layered structure with alternating "apatite layers" similar to HA and "hydrated layers" containing water molecules and loosely bound ions [, ]. In contrast, HA has a more compact, hexagonal structure.
Q4: Does the presence of carbonate ions affect the properties of this compound?
A4: Yes, the incorporation of carbonate ions into the this compound (OCP) lattice can enhance bone regeneration. Studies have shown that calcium-deficient carbonate apatite, a product of OCP hydrolysis, stimulated osteoblast differentiation more effectively than its carbonate-free counterpart, calcium-deficient hydroxyapatite [, ].
Q5: How does this compound interact with biological fluids like simulated body fluid (SBF)?
A5: OCP undergoes dissolution and precipitation reactions when exposed to simulated body fluid (SBF) []. This process often leads to the formation of a hydroxyapatite (HA) layer on the OCP surface, indicating its transformation towards a more stable phase in physiological conditions [].
Q6: Is this compound stable in physiological conditions?
A6: OCP is considered metastable under physiological conditions []. It tends to hydrolyze into the more stable hydroxyapatite (HA) []. This transformation is influenced by factors like pH, the presence of ions, and organic molecules.
Q7: Does the preparation method of this compound influence its properties and interaction with biomolecules?
A7: Yes, studies have shown that the method of OCP preparation can influence its properties, such as surface area and morphology, which in turn affect biomolecule adsorption []. For example, OCP prepared in the presence of gelatin exhibited lower bovine serum albumin adsorption compared to OCP prepared without gelatin [].
Q8: How does this compound promote bone regeneration?
A8: OCP exhibits osteoconductive properties, meaning it acts as a scaffold for new bone growth []. Its transformation into HA in vivo further contributes to bone regeneration. Studies show that OCP enhances bone regeneration compared to HA alone [, , , ].
Q9: Can this compound be used in combination with other materials to enhance its bone regeneration potential?
A9: Yes, OCP is often combined with other materials like collagen to create composites with improved handling and mechanical properties for bone regeneration [, , , , , ]. These composites demonstrated superior bone regeneration compared to OCP or collagen alone in various animal models [].
Q10: How does this compound interact with osteoblasts, the cells responsible for bone formation?
A10: OCP stimulates the differentiation of osteoblasts, enhancing their bone-forming activity []. This is attributed to the release of phosphate ions during its conversion to HA, which act as signaling molecules for osteoblast differentiation [].
Q11: What are the advantages of using this compound over other calcium phosphate ceramics like β-tricalcium phosphate in bone tissue engineering?
A11: While β-tricalcium phosphate boasts good biocompatibility, OCP exhibits superior osteoconductivity and osteoinductivity, making it more appealing for bone tissue engineering []. Combining OCP with other biocompatible materials like β-tricalcium phosphate can lead to novel biomaterials with enhanced biological performance [].
Q12: Are there any potential applications of this compound beyond bone regeneration?
A12: Research suggests that OCP could have applications in drug delivery systems due to its ability to be loaded with various ions and molecules []. For example, studies have explored its potential as a carrier for growth factors to further enhance bone regeneration [].
Q13: Can this compound be used as a coating material for metallic implants?
A13: Yes, OCP can be deposited onto metallic implant surfaces to improve biocompatibility and osseointegration [, , ]. This can be achieved through methods like biomimetic deposition and electrochemical deposition [, ]. The presence of the OCP coating can enhance cell attachment, proliferation, and differentiation, promoting better integration of the implant with the surrounding bone tissue [].
Q14: Can the properties of this compound be tailored for specific applications?
A14: Yes, the properties of OCP can be tailored by incorporating different ions, such as magnesium, into its structure []. Magnesium substitution can alter the crystal lattice parameters and particle size of OCP, potentially influencing its solubility and biodegradation rate []. This ability to modulate its properties makes Mg-doped OCP promising for applications like bone implants and cements [].
Disclaimer and Information on In-Vitro Research Products
Please be aware that all articles and product information presented on BenchChem are intended solely for informational purposes. The products available for purchase on BenchChem are specifically designed for in-vitro studies, which are conducted outside of living organisms. In-vitro studies, derived from the Latin term "in glass," involve experiments performed in controlled laboratory settings using cells or tissues. It is important to note that these products are not categorized as medicines or drugs, and they have not received approval from the FDA for the prevention, treatment, or cure of any medical condition, ailment, or disease. We must emphasize that any form of bodily introduction of these products into humans or animals is strictly prohibited by law. It is essential to adhere to these guidelines to ensure compliance with legal and ethical standards in research and experimentation.