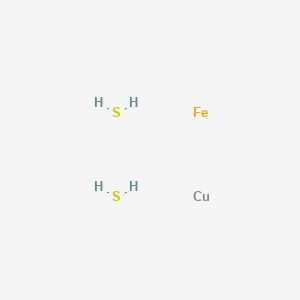
Copper;iron;sulfane
- Click on QUICK INQUIRY to receive a quote from our team of experts.
- With the quality product at a COMPETITIVE price, you can focus more on your research.
Overview
Description
Copper;iron;sulfane, commonly known as chalcopyrite, is a copper iron sulfide mineral with the chemical formula CuFeS₂. It is the most abundant copper ore mineral and has a brassy to golden yellow color. Chalcopyrite crystallizes in the tetragonal system and has a hardness of 3.5 to 4 on the Mohs scale .
Preparation Methods
Synthetic Routes and Reaction Conditions: Chalcopyrite can be synthesized using various methods, including pyrometallurgy and hydrometallurgy. In pyrometallurgy, copper and iron sulfides are heated in the presence of oxygen to produce copper and iron oxides, which are then reduced to obtain the metal. Hydrometallurgy involves leaching chalcopyrite with acidic or basic solutions to extract copper and iron .
Industrial Production Methods: Industrial production of chalcopyrite involves mining the ore from deposits, followed by crushing and grinding to liberate the mineral particles. The ore is then concentrated using froth flotation, where chalcopyrite is separated from other minerals. The concentrated chalcopyrite is further processed using pyrometallurgical or hydrometallurgical methods to extract copper and iron .
Chemical Reactions Analysis
Types of Reactions: Chalcopyrite undergoes various chemical reactions, including oxidation, reduction, and substitution.
Common Reagents and Conditions:
Oxidation: Chalcopyrite reacts with oxygen to form copper and iron oxides. For example, heating chalcopyrite in the presence of oxygen produces copper(II) oxide and iron(III) oxide.
Reduction: Chalcopyrite can be reduced using carbon monoxide or hydrogen to produce metallic copper and iron.
Substitution: Chalcopyrite can react with other metal sulfides to form different compounds.
Major Products Formed:
Oxidation: Copper(II) oxide (CuO) and iron(III) oxide (Fe₂O₃).
Reduction: Metallic copper (Cu) and iron (Fe).
Substitution: Copper sulfide (CuS) and zinc iron sulfide (ZnFeS) .
Scientific Research Applications
Chalcopyrite has numerous scientific research applications, including:
Chemistry: Chalcopyrite is used as a source of copper and iron in various chemical reactions and processes. It is also studied for its unique crystal structure and electronic properties.
Biology: Chalcopyrite nanoparticles are explored for their potential use in biomedical applications, such as drug delivery and imaging.
Medicine: Chalcopyrite-based materials are investigated for their antimicrobial properties and potential use in medical devices.
Mechanism of Action
The mechanism of action of chalcopyrite involves its interaction with various molecular targets and pathways. In biological systems, chalcopyrite nanoparticles can generate reactive oxygen species (ROS) through redox reactions, leading to oxidative stress and cell damage. This property is exploited in antimicrobial applications, where chalcopyrite nanoparticles can kill bacteria by inducing oxidative stress .
In industrial processes, chalcopyrite undergoes redox reactions to produce copper and iron. The copper ions in chalcopyrite can participate in electron transfer reactions, making it a valuable material for catalytic applications .
Comparison with Similar Compounds
Chalcopyrite is unique among copper iron sulfide minerals due to its specific chemical composition and crystal structure. Similar compounds include:
Bornite (Cu₅FeS₄): Another copper iron sulfide mineral with a different stoichiometry and crystal structure.
Chalcocite (Cu₂S): A copper sulfide mineral with no iron content.
Covellite (CuS): A copper sulfide mineral with a different crystal structure and properties.
Chalcopyrite is distinguished by its tetragonal crystal structure and its role as the most abundant copper ore mineral. Its unique combination of copper, iron, and sulfur atoms gives it distinct electronic and catalytic properties .
Properties
CAS No. |
1308-56-1 |
---|---|
Molecular Formula |
CuFeH2S |
Molecular Weight |
153.47 g/mol |
IUPAC Name |
copper;iron;sulfane |
InChI |
InChI=1S/Cu.Fe.H2S/h;;1H2 |
InChI Key |
BUGICWZUDIWQRQ-UHFFFAOYSA-N |
SMILES |
S.S.[Fe].[Cu] |
Canonical SMILES |
S.[Fe].[Cu] |
Key on ui other cas no. |
1308-56-1 |
Origin of Product |
United States |
Disclaimer and Information on In-Vitro Research Products
Please be aware that all articles and product information presented on BenchChem are intended solely for informational purposes. The products available for purchase on BenchChem are specifically designed for in-vitro studies, which are conducted outside of living organisms. In-vitro studies, derived from the Latin term "in glass," involve experiments performed in controlled laboratory settings using cells or tissues. It is important to note that these products are not categorized as medicines or drugs, and they have not received approval from the FDA for the prevention, treatment, or cure of any medical condition, ailment, or disease. We must emphasize that any form of bodily introduction of these products into humans or animals is strictly prohibited by law. It is essential to adhere to these guidelines to ensure compliance with legal and ethical standards in research and experimentation.