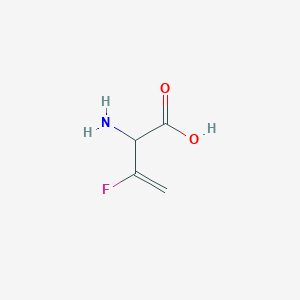
Fluorovinylglycine
Overview
Description
3-Fluorovinylglycine is a fluorinated amino acid derivative that has garnered interest due to its unique chemical properties and potential applications in various fields. This compound is characterized by the presence of a fluorovinyl group attached to the glycine backbone, which imparts distinct reactivity and biological activity.
Preparation Methods
Synthetic Routes and Reaction Conditions
The synthesis of 3-fluorovinylglycine typically involves the use of 2-fluoroacrolein as a starting material. One common method includes the reaction of 2-fluoroacrolein with 4,4’-dimethoxybenzhydrylamine to form an intermediate, which is then hydrolyzed to yield racemic 3-fluorovinylglycine . Another approach involves the catalytic isomerization of tetrafluoroethane-β-sultone, which is obtained by reacting tetrafluoroethylene with sulfur trioxide .
Industrial Production Methods
Industrial production methods for 3-fluorovinylglycine are not extensively documented, but the synthetic routes mentioned above can be scaled up for larger-scale production. The choice of method depends on factors such as yield, purity, and cost-effectiveness.
Chemical Reactions Analysis
Types of Reactions
3-Fluorovinylglycine undergoes various chemical reactions, including:
Oxidation: The compound can be oxidized under specific conditions to form corresponding oxides.
Reduction: Reduction reactions can convert the fluorovinyl group to other functional groups.
Substitution: The fluorovinyl group can participate in substitution reactions, where the fluorine atom is replaced by other substituents.
Common Reagents and Conditions
Oxidation: Common oxidizing agents include potassium permanganate and hydrogen peroxide.
Reduction: Reducing agents such as lithium aluminum hydride and sodium borohydride are used.
Substitution: Nucleophiles like amines and thiols can be used for substitution reactions.
Major Products Formed
The major products formed from these reactions depend on the specific reagents and conditions used. For example, oxidation may yield fluorinated carboxylic acids, while reduction can produce fluorinated alcohols.
Scientific Research Applications
3-Fluorovinylglycine has several scientific research applications:
Chemistry: It is used as a building block in the synthesis of complex molecules with potential biological activity.
Medicine: Its potential as an antimicrobial agent is being explored due to its enzyme inhibition properties.
Industry: The compound’s unique reactivity makes it useful in the development of new materials and pharmaceuticals.
Mechanism of Action
The mechanism of action of 3-fluorovinylglycine involves the inhibition of enzymes such as alanine racemase. The compound undergoes enzyme-catalyzed halide elimination to form an allenic intermediate, which partitions between reversible and irreversible covalent adducts . The irreversible adduct involves the alkylation of a tyrosine residue in the enzyme, leading to its inactivation .
Comparison with Similar Compounds
3-Fluorovinylglycine can be compared with other halovinylglycines such as 3-chlorovinylglycine and 3-bromovinylglycine. These compounds share similar structures but differ in their halogen substituents, which affect their reactivity and biological activity . For example, 3-chlorovinylglycine has a higher second-order rate constant for enzyme inhibition compared to 3-fluorovinylglycine .
List of Similar Compounds
- 3-Chlorovinylglycine
- 3-Bromovinylglycine
- Fluoroalanine
These compounds highlight the unique properties of 3-fluorovinylglycine, particularly its balance between reactivity and stability.
Biological Activity
Fluorovinylglycine (FVG) is an amino acid analog that has garnered attention due to its potential biological activities, particularly as an inhibitor of key enzymes in bacterial metabolism. This article delves into the biological activity of this compound, focusing on its mechanisms of action, antibacterial properties, and implications for drug development.
Chemical Structure and Synthesis
This compound is characterized by the presence of a fluorinated vinyl group attached to the glycine backbone. The synthesis of FVG typically involves the introduction of fluorinated olefins into amino acid structures, which can significantly alter their biochemical properties. Recent studies have explored various synthetic pathways to produce FVG and its derivatives, revealing insights into their reactivity and stability.
This compound acts primarily as a mechanism-based inhibitor of the enzyme alanine racemase (Alr), which is crucial for bacterial cell wall synthesis. Alr catalyzes the conversion of L-alanine to D-alanine, a necessary component for peptidoglycan biosynthesis in bacteria.
- Inhibition Studies : Research indicates that both enantiomers of this compound exhibit potent inhibitory effects on Alr. For instance, studies have shown that 3-fluorovinylglycine irreversibly inactivates Alr in various bacterial strains, including Staphylococcus aureus and Pseudomonas aeruginosa .
- Binding Mechanism : The binding of FVG to Alr leads to a conformational change in the enzyme, ultimately preventing substrate access and inhibiting enzymatic activity. This mechanism is particularly effective against Gram-positive bacteria, highlighting FVG's potential as an antimicrobial agent.
Antibacterial Activity
The antibacterial efficacy of this compound has been assessed through various studies:
- Minimum Inhibitory Concentration (MIC) : The MIC values for FVG derivatives against several bacterial strains have been reported, demonstrating significant antibacterial activity. For example, L-norvalyl-L-fluorovinylglycine showed promising results against methicillin-resistant Staphylococcus aureus (MRSA) with an MIC comparable to traditional antibiotics .
- Comparative Studies : Comparative analyses with other fluorinated amino acids have shown that FVG derivatives often exhibit superior antibacterial properties due to their enhanced ability to inhibit Alr and disrupt bacterial cell wall synthesis .
Case Studies
Several case studies highlight the practical applications and effectiveness of this compound:
- Study on Gram-positive Bacteria : A study demonstrated that 3-fluorovinylglycine effectively inhibited growth in Gram-positive bacteria, with a particular focus on its action against MRSA. The results indicated a strong correlation between the concentration of FVG and the degree of bacterial inhibition .
- Mechanistic Insights : Another research effort provided detailed mechanistic insights into how FVG binds to Alr, revealing that it forms a stable complex that prevents enzyme activity. This study utilized kinetic assays and structural analysis to elucidate the binding interactions .
Research Findings Summary
Compound | Target Enzyme | Activity | MIC (µg/mL) | Bacterial Strain |
---|---|---|---|---|
3-Fluorovinylglycine | Alanine Racemase | Inhibitor | 8-16 | MRSA |
L-Norvalyl-L-Fluorovinylglycine | Alanine Racemase | Inhibitor | 4-8 | Pseudomonas aeruginosa |
3-Chlorovinylglycine | Alanine Racemase | Inhibitor | 2-4 | Streptococcus faecalis |
Properties
IUPAC Name |
2-amino-3-fluorobut-3-enoic acid | |
---|---|---|
Source | PubChem | |
URL | https://pubchem.ncbi.nlm.nih.gov | |
Description | Data deposited in or computed by PubChem | |
InChI |
InChI=1S/C4H6FNO2/c1-2(5)3(6)4(7)8/h3H,1,6H2,(H,7,8) | |
Source | PubChem | |
URL | https://pubchem.ncbi.nlm.nih.gov | |
Description | Data deposited in or computed by PubChem | |
InChI Key |
HMOXWHQSNHKKFM-UHFFFAOYSA-N | |
Source | PubChem | |
URL | https://pubchem.ncbi.nlm.nih.gov | |
Description | Data deposited in or computed by PubChem | |
Canonical SMILES |
C=C(C(C(=O)O)N)F | |
Source | PubChem | |
URL | https://pubchem.ncbi.nlm.nih.gov | |
Description | Data deposited in or computed by PubChem | |
Molecular Formula |
C4H6FNO2 | |
Source | PubChem | |
URL | https://pubchem.ncbi.nlm.nih.gov | |
Description | Data deposited in or computed by PubChem | |
DSSTOX Substance ID |
DTXSID30912228 | |
Record name | 2-Amino-3-fluorobut-3-enoic acid | |
Source | EPA DSSTox | |
URL | https://comptox.epa.gov/dashboard/DTXSID30912228 | |
Description | DSSTox provides a high quality public chemistry resource for supporting improved predictive toxicology. | |
Molecular Weight |
119.09 g/mol | |
Source | PubChem | |
URL | https://pubchem.ncbi.nlm.nih.gov | |
Description | Data deposited in or computed by PubChem | |
CAS No. |
111581-52-3 | |
Record name | Fluorovinylglycine | |
Source | ChemIDplus | |
URL | https://pubchem.ncbi.nlm.nih.gov/substance/?source=chemidplus&sourceid=0111581523 | |
Description | ChemIDplus is a free, web search system that provides access to the structure and nomenclature authority files used for the identification of chemical substances cited in National Library of Medicine (NLM) databases, including the TOXNET system. | |
Record name | 2-Amino-3-fluorobut-3-enoic acid | |
Source | EPA DSSTox | |
URL | https://comptox.epa.gov/dashboard/DTXSID30912228 | |
Description | DSSTox provides a high quality public chemistry resource for supporting improved predictive toxicology. | |
Retrosynthesis Analysis
AI-Powered Synthesis Planning: Our tool employs the Template_relevance Pistachio, Template_relevance Bkms_metabolic, Template_relevance Pistachio_ringbreaker, Template_relevance Reaxys, Template_relevance Reaxys_biocatalysis model, leveraging a vast database of chemical reactions to predict feasible synthetic routes.
One-Step Synthesis Focus: Specifically designed for one-step synthesis, it provides concise and direct routes for your target compounds, streamlining the synthesis process.
Accurate Predictions: Utilizing the extensive PISTACHIO, BKMS_METABOLIC, PISTACHIO_RINGBREAKER, REAXYS, REAXYS_BIOCATALYSIS database, our tool offers high-accuracy predictions, reflecting the latest in chemical research and data.
Strategy Settings
Precursor scoring | Relevance Heuristic |
---|---|
Min. plausibility | 0.01 |
Model | Template_relevance |
Template Set | Pistachio/Bkms_metabolic/Pistachio_ringbreaker/Reaxys/Reaxys_biocatalysis |
Top-N result to add to graph | 6 |
Feasible Synthetic Routes
Disclaimer and Information on In-Vitro Research Products
Please be aware that all articles and product information presented on BenchChem are intended solely for informational purposes. The products available for purchase on BenchChem are specifically designed for in-vitro studies, which are conducted outside of living organisms. In-vitro studies, derived from the Latin term "in glass," involve experiments performed in controlled laboratory settings using cells or tissues. It is important to note that these products are not categorized as medicines or drugs, and they have not received approval from the FDA for the prevention, treatment, or cure of any medical condition, ailment, or disease. We must emphasize that any form of bodily introduction of these products into humans or animals is strictly prohibited by law. It is essential to adhere to these guidelines to ensure compliance with legal and ethical standards in research and experimentation.