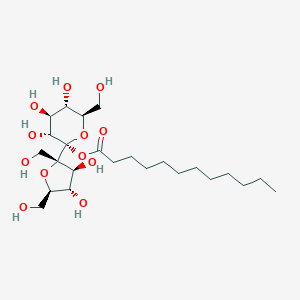
Sucrose laurate
- Click on QUICK INQUIRY to receive a quote from our team of experts.
- With the quality product at a COMPETITIVE price, you can focus more on your research.
Overview
Description
Sucrose laurate is a type of sucrose ester, which is a non-ionic surfactant. It is formed by the esterification of sucrose with lauric acid. This compound is widely used in various industries due to its excellent emulsifying, stabilizing, and surfactant properties. It is commonly found in food, cosmetics, and pharmaceutical products.
Mechanism of Action
Target of Action
Sucrose laurate, also known as [(2S,3R,4S,5S,6R)-2-[(2S,3S,4S,5R)-3,4-dihydroxy-2,5-bis(hydroxymethyl)oxolan-2-yl]-3,4,5-trihydroxy-6-(hydroxymethyl)oxan-2-yl] dodecanoate, primarily targets the intestinal epithelial cells . It interacts with the plasma membrane and tight junction proteins of these cells, such as ZO-1 .
Mode of Action
This compound acts as a permeation enhancer (PE), working to reversibly enhance epithelial permeability via the paracellular route . It increases the apparent permeability coefficient (Papp) of certain substances and reduces transepithelial electrical resistance (TEER) across monolayers . This compound alters the expression of the tight junction protein, ZO-1, increases plasma membrane potential, and decreases mitochondrial membrane potential in Caco-2 cells .
Biochemical Pathways
This compound affects multiple biochemical pathways. It has been found to down-regulate cell wall-associated hydrolase, inhibit the synthesis of fatty acids, influence nucleic acid synthesis, disturb amino acid metabolism, and block the HMP pathway and TCA cycle .
Pharmacokinetics
This compound’s ADME properties significantly impact its bioavailability. In rat jejunal and colonic instillations, 50 and 100 mM this compound co-administered with insulin induced blood glucose reductions and achieved relative bioavailability values of 2.4% and 8.9%, respectively . This is on par with the gold standard PE, sodium caprate (C10) .
Result of Action
The primary result of this compound’s action is plasma membrane perturbation, leading to tight junction openings and a predominant paracellular flux . This results in increased permeability and enhanced absorption of certain substances, including macromolecules . It also causes the leakage of intracellular materials .
Action Environment
The action of this compound can be influenced by environmental factors. For instance, the concentration of this compound plays a crucial role in its efficacy . Higher concentrations can induce cytotoxicity, while lower concentrations can enhance permeability . Furthermore, temperature can affect the production yield and selectivity of this compound .
Biochemical Analysis
Biochemical Properties
Sucrose laurate plays a key role in biochemical reactions. It acts as an emulsifier, enabling the mixing of water and oil-based components in cosmetics . It also serves as a skin conditioning agent, an emollient, and a cleanser . In the pharmaceutical industry, it aids in the solubilization and absorption of active pharmaceutical ingredients, ensuring their effectiveness . Moreover, it can enhance the bioavailability of poorly soluble drugs, making them more readily absorbed by the body .
Cellular Effects
This compound has various effects on different types of cells and cellular processes. For instance, it has been shown to increase the apparent permeability coefficient (Papp) of [14C]-mannitol and reduce transepithelial electrical resistance (TEER) across Caco-2 monolayers . It alters the expression of the tight junction protein, ZO-1, increases plasma membrane potential, and decreases mitochondrial membrane potential in Caco-2 cells .
Molecular Mechanism
The primary action of this compound is plasma membrane perturbation, leading to tight junction openings and a predominant paracellular flux . This mechanism allows this compound to effectively lift away dirt, makeup, and excess sebum without disrupting the skin’s natural lipid barrier .
Dosage Effects in Animal Models
The effects of this compound vary with different dosages in animal models. For instance, in rat jejunal and colonic instillations, 50 and 100 mM this compound co-administered with insulin induced blood glucose reductions .
Metabolic Pathways
This compound is involved in the esterification reaction where sugar is reacted with free fatty acid under a homogenous acid catalyst to produce sugar ester and water . This process is generally carried out under reduced pressure and anhydrous conditions .
Transport and Distribution
Its ability to act as an emulsifier, enabling the mixing of water and oil-based components, suggests that it may be distributed in both hydrophilic and lipophilic environments .
Subcellular Localization
Given its role as an emulsifier and its impact on cellular processes such as the disruption of tight junctions , it is likely that this compound interacts with various cellular compartments.
Preparation Methods
Synthetic Routes and Reaction Conditions: Sucrose laurate can be synthesized through a two-stage process. In the first stage, lauric acid is esterified with methanol to produce methyl laurate. This reaction is typically catalyzed by Amberlyst 15, a strong acid ion-exchange resin, at a temperature of 110°C with a residence time of 5 minutes . In the second stage, methyl laurate undergoes transesterification with sucrose to form this compound. This process is carried out under reduced pressure and anhydrous conditions to achieve high selectivity and yield .
Industrial Production Methods: Industrial production of this compound often involves the use of ultrasonic frequencies to enhance the reaction efficiency. Ultrasonic waves can reduce reaction temperature and pressure, improve yield, and shorten reaction time . The process involves the transesterification of sucrose with lauric acid or its esters under ultrasonic conditions, resulting in a mixture of sucrose monolaurate, dilaurate, and polylaurate .
Chemical Reactions Analysis
Types of Reactions: Sucrose laurate primarily undergoes esterification and transesterification reactions. It can also participate in hydrolysis and saponification reactions under certain conditions .
Common Reagents and Conditions:
Esterification: Lauric acid and methanol with Amberlyst 15 as a catalyst at 110°C.
Transesterification: Methyl laurate and sucrose under reduced pressure and anhydrous conditions.
Hydrolysis and Saponification: These reactions typically occur in the presence of water and a base, such as sodium hydroxide.
Major Products:
Esterification: Methyl laurate.
Transesterification: Sucrose monolaurate, dilaurate, and polylaurate.
Hydrolysis and Saponification: Lauric acid and sucrose.
Scientific Research Applications
Sucrose laurate has a wide range of applications in scientific research:
Comparison with Similar Compounds
Sucrose laurate is compared with other sucrose esters such as sucrose stearate and sucrose palmitate. While all these compounds are used as surfactants and emulsifiers, this compound is unique in its ability to enhance intestinal permeability and facilitate drug absorption . Similar compounds include:
Sucrose stearate: Used in cosmetics and pharmaceuticals for its emulsifying properties.
Sucrose palmitate: Commonly used in food products as an emulsifier.
This compound stands out due to its specific applications in drug delivery and its effectiveness as an intestinal permeation enhancer .
Properties
CAS No. |
25339-99-5 |
---|---|
Molecular Formula |
C24H46O13 |
Molecular Weight |
542.6 g/mol |
IUPAC Name |
2-[(2S)-3,4-dihydroxy-2,5-bis(hydroxymethyl)oxolan-2-yl]oxy-6-(hydroxymethyl)oxane-3,4,5-triol;dodecanoic acid |
InChI |
InChI=1S/C12H22O11.C12H24O2/c13-1-4-6(16)8(18)9(19)11(21-4)23-12(3-15)10(20)7(17)5(2-14)22-12;1-2-3-4-5-6-7-8-9-10-11-12(13)14/h4-11,13-20H,1-3H2;2-11H2,1H3,(H,13,14)/t4?,5?,6?,7?,8?,9?,10?,11?,12-;/m0./s1 |
InChI Key |
PVVVEHXCVQRLOC-NSIFZURYSA-N |
SMILES |
CCCCCCCCCCCC(=O)OC1(C(C(C(C(O1)CO)O)O)O)C2(C(C(C(O2)CO)O)O)CO |
Isomeric SMILES |
CCCCCCCCCCCC(=O)O.C(C1C(C(C(C(O1)O[C@]2(C(C(C(O2)CO)O)O)CO)O)O)O)O |
Canonical SMILES |
CCCCCCCCCCCC(=O)O.C(C1C(C(C(C(O1)OC2(C(C(C(O2)CO)O)O)CO)O)O)O)O |
Key on ui other cas no. |
25339-99-5 |
Pictograms |
Corrosive |
Related CAS |
37266-93-6 (unspecified laurate) |
Synonyms |
eta-fructofuranosyl-alpha-D-glucopyranoside monododecanoate L-1690 sucrose laurate sucrose monododecanoate sucrose monolaurate |
Origin of Product |
United States |
Disclaimer and Information on In-Vitro Research Products
Please be aware that all articles and product information presented on BenchChem are intended solely for informational purposes. The products available for purchase on BenchChem are specifically designed for in-vitro studies, which are conducted outside of living organisms. In-vitro studies, derived from the Latin term "in glass," involve experiments performed in controlled laboratory settings using cells or tissues. It is important to note that these products are not categorized as medicines or drugs, and they have not received approval from the FDA for the prevention, treatment, or cure of any medical condition, ailment, or disease. We must emphasize that any form of bodily introduction of these products into humans or animals is strictly prohibited by law. It is essential to adhere to these guidelines to ensure compliance with legal and ethical standards in research and experimentation.