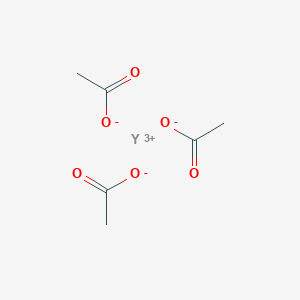
Yttrium acetate
Overview
Description
Yttrium acetate is a chemical compound with the formula (CH₃CO₂)₃Y. It is a white, crystalline salt that is soluble in water and mineral acids. This compound is commonly used as a precursor in the synthesis of other yttrium compounds, including yttrium oxides and yttrium fluorides .
Mechanism of Action
Target of Action
Yttrium acetate, a compound of the rare earth element yttrium, primarily targets calcium-dependent processes in vertebrate myocardium . It also finds use in medical imaging and therapy, particularly in the form of its isotopes, such as 90Y complexes for radiopharmaceuticals .
Mode of Action
This compound interacts with its targets by substituting calcium ions in biological systems. It has been found to decrease the rate of heart contractions and stimulate ion transport in rat heart mitochondria . This compound induces inhibition of energy-dependent Ca2+ transport into mitochondria, which results in a marked decrease of their swelling .
Biochemical Pathways
The action of this compound affects the calcium-dependent biochemical pathways in the myocardium. It inhibits the potential-modulated Ca2±channels of pacemaker and contractile cardiomyocytes (CM), and also affects the Ca2±carrier in the inner mitochondrial membrane (IMM) . The data confirming that Y3+ activates energy-dependent K+ transport catalyzed by mitochondrial uniporter and blocks Ca2±channels in the mitochondrial membrane are important for understanding the mechanisms of the Y3+ action on vertebrates and human CM .
Pharmacokinetics
The pharmacokinetics of yttrium, particularly in the form of its isotopes, has been studied extensively. For instance, the radioactive properties of 177Lu and 90Y, including their plasma and whole-body clearance kinetics, are similar . The terminal half-life is approximately 44 hours for both isotopes . The liver, bone, and spleen are the primary organs where yttrium is distributed .
Result of Action
The action of this compound results in molecular and cellular effects, particularly in the myocardium. It decreases the rate of heart contractions and stimulates ion transport in rat heart mitochondria . It also inhibits energy-dependent Ca2+ transport into mitochondria, affecting their swelling .
Action Environment
The action, efficacy, and stability of this compound can be influenced by environmental factors. For instance, the solubility of this compound in water and mineral acids, as well as in solutions that complex with the Y3+ cations, can affect its action . Moreover, the temperature can influence the reaction of this compound, particularly in the synthesis of sodium yttrium fluoride (NaYF4) nanoparticles .
Biochemical Analysis
Biochemical Properties
Yttrium acetate can interact with various biomolecules. Due to its chemical similarities, yttrium acts like a lanthanide in respect to its toxicological behavior
Cellular Effects
This compound can have various effects on cells. For instance, studies have shown that yttrium chloride, a related compound, can cause acute hepatic injury and a transient increase of plasma calcium
Temporal Effects in Laboratory Settings
In laboratory settings, this compound’s effects can change over time. For example, studies on yttrium chloride have shown that it is taken up by phagocytic cells in the liver and spleen, and the elimination half-time of liver yttrium was 144 days .
Dosage Effects in Animal Models
The effects of this compound in animal models can vary with dosage. While specific studies on this compound are limited, related compounds have shown dose-dependent effects .
Transport and Distribution
This compound can be transported and distributed within cells and tissues. Studies on yttrium chloride have shown that it is taken up by phagocytic cells in the liver and spleen .
Subcellular Localization
Studies on yttrium chloride have shown that it is taken up by phagocytic cells in the liver and spleen .
Preparation Methods
Synthetic Routes and Reaction Conditions: Yttrium acetate can be synthesized by reacting yttrium oxide with acetic acid. The process involves the following steps :
- Pump analytically-pure glacial acetic acid and deionized water into a reaction kettle in a 1:1 volume ratio.
- Stir and heat the mixture until it boils.
- Slowly add yttrium oxide to the reaction kettle while rotating the stirring paddle at a speed of 60 to 80 revolutions per minute.
- After the reaction is complete, heat the liquid to 110-125°C, then allow it to cool and crystallize naturally.
- Filter the liquid, centrifugally dewater the reactant, and air the dewatered product in a dust-free room to achieve natural evaporation.
Industrial Production Methods: The industrial production of this compound follows a similar process but on a larger scale. The method is designed to be simple, efficient, and environmentally friendly, reducing production costs and pollution .
Chemical Reactions Analysis
Types of Reactions: Yttrium acetate undergoes various chemical reactions, including:
Oxidation: this compound can be oxidized to form yttrium oxide.
Reduction: It can be reduced to form yttrium metal.
Substitution: this compound can react with other acids to form different yttrium salts.
Common Reagents and Conditions:
Oxidation: Heating this compound in the presence of oxygen.
Reduction: Using reducing agents such as hydrogen gas.
Substitution: Reacting with acids like hydrochloric acid or sulfuric acid.
Major Products:
Oxidation: Yttrium oxide (Y₂O₃).
Reduction: Yttrium metal (Y).
Substitution: Yttrium chloride (YCl₃), yttrium sulfate (Y₂(SO₄)₃).
Scientific Research Applications
Yttrium acetate has a wide range of applications in scientific research :
Chemistry: Used as a precursor for synthesizing yttrium-based materials, including yttrium oxides and yttrium fluorides.
Biology: Utilized in the synthesis of nanoparticles for biological imaging and sensing.
Medicine: Employed in medical imaging and therapy, including positron emission tomography (PET) imaging and radiotherapy.
Industry: Used in the production of high-purity yttrium compounds for various industrial applications, such as ceramics, phosphors, and fuel sensors
Comparison with Similar Compounds
Yttrium acetate is unique due to its high solubility in water and low thermal decomposition temperature, making it attractive for hydrothermal reactions and co-precipitation processing . Similar compounds include:
- Yttrium formate (Y(HCOO)₃)
- Yttrium propionate (Y(C₂H₅COO)₃)
- Yttrium butyrate (Y(C₃H₇COO)₃)
These compounds share similar properties but differ in their solubility and thermal stability.
Properties
CAS No. |
23363-14-6 |
---|---|
Molecular Formula |
C6H12O6Y |
Molecular Weight |
269.06 g/mol |
IUPAC Name |
acetic acid;yttrium |
InChI |
InChI=1S/3C2H4O2.Y/c3*1-2(3)4;/h3*1H3,(H,3,4); |
InChI Key |
NRCXXAZNACNPRH-UHFFFAOYSA-N |
SMILES |
CC(=O)[O-].CC(=O)[O-].CC(=O)[O-].[Y+3] |
Canonical SMILES |
CC(=O)O.CC(=O)O.CC(=O)O.[Y] |
Key on ui other cas no. |
23363-14-6 |
physical_description |
Pellets or Large Crystals Nonahydrate: Colorless solid; [Hawley] Hydrate: White crystals; [MSDSonline] |
Pictograms |
Irritant |
Origin of Product |
United States |
Synthesis routes and methods I
Procedure details
Synthesis routes and methods II
Procedure details
Synthesis routes and methods III
Procedure details
Retrosynthesis Analysis
AI-Powered Synthesis Planning: Our tool employs the Template_relevance Pistachio, Template_relevance Bkms_metabolic, Template_relevance Pistachio_ringbreaker, Template_relevance Reaxys, Template_relevance Reaxys_biocatalysis model, leveraging a vast database of chemical reactions to predict feasible synthetic routes.
One-Step Synthesis Focus: Specifically designed for one-step synthesis, it provides concise and direct routes for your target compounds, streamlining the synthesis process.
Accurate Predictions: Utilizing the extensive PISTACHIO, BKMS_METABOLIC, PISTACHIO_RINGBREAKER, REAXYS, REAXYS_BIOCATALYSIS database, our tool offers high-accuracy predictions, reflecting the latest in chemical research and data.
Strategy Settings
Precursor scoring | Relevance Heuristic |
---|---|
Min. plausibility | 0.01 |
Model | Template_relevance |
Template Set | Pistachio/Bkms_metabolic/Pistachio_ringbreaker/Reaxys/Reaxys_biocatalysis |
Top-N result to add to graph | 6 |
Feasible Synthetic Routes
Disclaimer and Information on In-Vitro Research Products
Please be aware that all articles and product information presented on BenchChem are intended solely for informational purposes. The products available for purchase on BenchChem are specifically designed for in-vitro studies, which are conducted outside of living organisms. In-vitro studies, derived from the Latin term "in glass," involve experiments performed in controlled laboratory settings using cells or tissues. It is important to note that these products are not categorized as medicines or drugs, and they have not received approval from the FDA for the prevention, treatment, or cure of any medical condition, ailment, or disease. We must emphasize that any form of bodily introduction of these products into humans or animals is strictly prohibited by law. It is essential to adhere to these guidelines to ensure compliance with legal and ethical standards in research and experimentation.