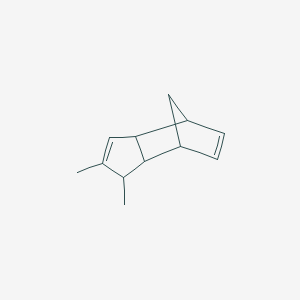
Methylcyclopentadiene dimer
Overview
Description
Methylcyclopentadiene dimer: is a chemical compound with the molecular formula C10H12 . It is a derivative of indene, characterized by its unique structure that includes a methano bridge and tetrahydro modifications.
Mechanism of Action
Target of Action
Dimethyldicyclopentadiene (DMDCPD), also known as 3a,4,7,7a-Tetrahydrodimethyl-4,7-methanoindene or 4,5-dimethyltricyclo[5.2.1.02,6]deca-3,8-diene, is primarily used in industrial applications, particularly in the production of high-performance jet fuels
Mode of Action
The primary mode of action of DMDCPD is through its chemical reactions. It is known to undergo Diels-Alder reactions easily, forming dimers and codimers with cyclopentadiene and its methyl derivatives .
Biochemical Pathways
Its formation involves thermal dimerization and codimerization reactions between cyclopentadiene and its methyl derivatives .
Pharmacokinetics
It’s important to note that it is slightly less dense than water and insoluble in water . This could potentially affect its distribution and elimination if it were to enter a biological system.
Result of Action
The main result of DMDCPD’s action is the formation of high-performance fuels when synthesized from 5-methyl furfural . These fuels exhibit high density and high thermal stability, superior to those of widely used fossil-based jet fuels such as JP-10 and JP-7 .
Action Environment
The action of DMDCPD is influenced by environmental factors such as temperature. The overall rates of cyclopentadiene and methylcyclopentadiene dimerizations and their mutual codimerization were found to be identical throughout the temperature range in which the reactions are kinetically controlled . Additionally, DMDCPD may react vigorously with strong oxidizing agents and can react exothermically with reducing agents to release gaseous hydrogen .
Preparation Methods
Synthetic Routes and Reaction Conditions: The synthesis of Methylcyclopentadiene dimer typically involves the dimerization of methylcyclopentadiene. This reaction is carried out under controlled conditions to ensure the formation of the desired product .
Industrial Production Methods: Industrial production of this compound involves the separation of methylcyclopentadiene from coal tar or cracked tar. The crude methylcyclopentadiene is then dimerized, followed by vacuum distillation to remove impurities and obtain the final product .
Chemical Reactions Analysis
Types of Reactions:
Oxidation: This compound can undergo oxidation reactions, often using reagents like potassium permanganate or chromium trioxide.
Reduction: Reduction reactions can be performed using hydrogen gas in the presence of a catalyst such as palladium on carbon.
Substitution: Substitution reactions, particularly electrophilic substitution, can occur with reagents like halogens or nitrating agents.
Common Reagents and Conditions:
Oxidation: Potassium permanganate in acidic or neutral medium.
Reduction: Hydrogen gas with palladium on carbon as a catalyst.
Substitution: Halogens (e.g., chlorine, bromine) in the presence of a Lewis acid catalyst.
Major Products Formed:
Oxidation: Formation of carboxylic acids or ketones.
Reduction: Formation of fully saturated hydrocarbons.
Substitution: Formation of halogenated or nitrated derivatives.
Scientific Research Applications
Chemistry: In chemistry, Methylcyclopentadiene dimer is used as a precursor for synthesizing more complex molecules. Its unique structure makes it a valuable intermediate in organic synthesis.
Biology and Medicine: In biology and medicine, this compound is explored for its potential therapeutic properties. It is studied for its role in drug development, particularly in designing molecules with specific biological activities .
Industry: In the industrial sector, this compound is used in the production of high-energy fuels, curing agents, plasticizers, resins, surface coatings, pharmaceuticals, and dyes .
Comparison with Similar Compounds
Indene: A parent compound with a simpler structure.
Tetrahydroindene: Similar but lacks the methano bridge.
Dimethylindene: Similar but lacks the tetrahydro modifications.
Uniqueness: Methylcyclopentadiene dimer is unique due to its combination of methano bridge and tetrahydro modifications, which confer distinct chemical and physical properties. This uniqueness makes it valuable in specialized applications where other similar compounds may not be as effective .
Properties
IUPAC Name |
4,5-dimethyltricyclo[5.2.1.02,6]deca-3,8-diene | |
---|---|---|
Source | PubChem | |
URL | https://pubchem.ncbi.nlm.nih.gov | |
Description | Data deposited in or computed by PubChem | |
InChI |
InChI=1S/C12H16/c1-7-5-11-9-3-4-10(6-9)12(11)8(7)2/h3-5,8-12H,6H2,1-2H3 | |
Source | PubChem | |
URL | https://pubchem.ncbi.nlm.nih.gov | |
Description | Data deposited in or computed by PubChem | |
InChI Key |
GUOAPVPPPVLIQQ-UHFFFAOYSA-N | |
Source | PubChem | |
URL | https://pubchem.ncbi.nlm.nih.gov | |
Description | Data deposited in or computed by PubChem | |
Canonical SMILES |
CC1C2C3CC(C2C=C1C)C=C3 | |
Source | PubChem | |
URL | https://pubchem.ncbi.nlm.nih.gov | |
Description | Data deposited in or computed by PubChem | |
Molecular Formula |
C12H16 | |
Source | PubChem | |
URL | https://pubchem.ncbi.nlm.nih.gov | |
Description | Data deposited in or computed by PubChem | |
DSSTOX Substance ID |
DTXSID80274242 | |
Record name | 4,7-Methano-1H-indene, 3a,4,7,7a-tetrahydro-1,2-dimethyl- | |
Source | EPA DSSTox | |
URL | https://comptox.epa.gov/dashboard/DTXSID80274242 | |
Description | DSSTox provides a high quality public chemistry resource for supporting improved predictive toxicology. | |
Molecular Weight |
160.25 g/mol | |
Source | PubChem | |
URL | https://pubchem.ncbi.nlm.nih.gov | |
Description | Data deposited in or computed by PubChem | |
CAS No. |
26472-00-4, 1217741-36-0 | |
Record name | Methylcyclopentadiene dimer | |
Source | ChemIDplus | |
URL | https://pubchem.ncbi.nlm.nih.gov/substance/?source=chemidplus&sourceid=0026472004 | |
Description | ChemIDplus is a free, web search system that provides access to the structure and nomenclature authority files used for the identification of chemical substances cited in National Library of Medicine (NLM) databases, including the TOXNET system. | |
Record name | 4,7-Methano-1H-indene, 3a,4,7,7a-tetrahydro-1,2-dimethyl- | |
Source | EPA DSSTox | |
URL | https://comptox.epa.gov/dashboard/DTXSID80274242 | |
Description | DSSTox provides a high quality public chemistry resource for supporting improved predictive toxicology. | |
Retrosynthesis Analysis
AI-Powered Synthesis Planning: Our tool employs the Template_relevance Pistachio, Template_relevance Bkms_metabolic, Template_relevance Pistachio_ringbreaker, Template_relevance Reaxys, Template_relevance Reaxys_biocatalysis model, leveraging a vast database of chemical reactions to predict feasible synthetic routes.
One-Step Synthesis Focus: Specifically designed for one-step synthesis, it provides concise and direct routes for your target compounds, streamlining the synthesis process.
Accurate Predictions: Utilizing the extensive PISTACHIO, BKMS_METABOLIC, PISTACHIO_RINGBREAKER, REAXYS, REAXYS_BIOCATALYSIS database, our tool offers high-accuracy predictions, reflecting the latest in chemical research and data.
Strategy Settings
Precursor scoring | Relevance Heuristic |
---|---|
Min. plausibility | 0.01 |
Model | Template_relevance |
Template Set | Pistachio/Bkms_metabolic/Pistachio_ringbreaker/Reaxys/Reaxys_biocatalysis |
Top-N result to add to graph | 6 |
Feasible Synthetic Routes
Q1: How can dimethyldicyclopentadiene be synthesized sustainably?
A2: A sustainable route for synthesizing dimethyldicyclopentadiene utilizes the bio-based platform chemical 2,5-hexanedione []. This three-step process involves:
- Aldol Condensation: 2,5-hexanedione undergoes a base-catalyzed intramolecular aldol condensation to yield 3-methyl-2-cyclopenten-1-one [].
- Chemoselective Hydrogenation: A ternary Ru catalyst system (RuCl2(PPh3)3/NH2(CH2)2NH2/KOH) facilitates the chemoselective reduction of 3-methyl-2-cyclopenten-1-one to 3-methyl-2-cyclopenten-1-ol with high selectivity (96%) [].
- Dehydration: Finally, dehydration of 3-methyl-2-cyclopenten-1-ol over AlPO4/MgSO4 at 70°C under reduced pressure yields methylcyclopentadiene []. Methylcyclopentadiene can then undergo a Diels-Alder reaction at ambient temperature to produce dimethyldicyclopentadiene [].
Q2: What is the significance of dimethyldicyclopentadiene in fuel applications?
A3: Dimethyldicyclopentadiene is a precursor to high-density fuels, particularly relevant for applications requiring high energy density, such as jet and missile propulsion []. For instance, it can be hydrogenated and isomerized to produce RJ-4, a high-density missile fuel [].
Q3: What are the analytical challenges associated with identifying minor dimethyldicyclopentadiene isomers in complex mixtures?
A4: Identifying minor dimethyldicyclopentadiene isomers in commercial mixtures presents a significant analytical challenge due to their low concentrations and the similarities in their chemical properties. Research has shown that fractional distillation combined with 13C NMR spectroscopy enables the identification of these minor isomers [], highlighting the need for advanced separation and analytical techniques.
Disclaimer and Information on In-Vitro Research Products
Please be aware that all articles and product information presented on BenchChem are intended solely for informational purposes. The products available for purchase on BenchChem are specifically designed for in-vitro studies, which are conducted outside of living organisms. In-vitro studies, derived from the Latin term "in glass," involve experiments performed in controlled laboratory settings using cells or tissues. It is important to note that these products are not categorized as medicines or drugs, and they have not received approval from the FDA for the prevention, treatment, or cure of any medical condition, ailment, or disease. We must emphasize that any form of bodily introduction of these products into humans or animals is strictly prohibited by law. It is essential to adhere to these guidelines to ensure compliance with legal and ethical standards in research and experimentation.