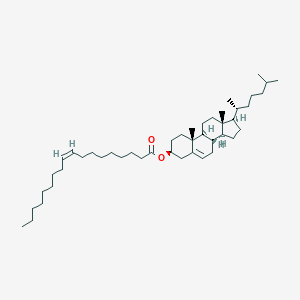
Cholesteryl oleate
Overview
Description
Cholesteryl oleate is a cholesteryl ester, which is an ester of cholesterol and oleic acid. It is a significant lipid molecule found in various biological systems, particularly in human plasma. This compound is known for its role in lipid metabolism and storage, and it is a major component of low-density lipoproteins (LDL) and high-density lipoproteins (HDL) in the bloodstream .
Mechanism of Action
Target of Action
Cholesteryl oleate primarily targets cholesterol metabolism within the body. It is an esterified form of cholesterol, where cholesterol is bonded to oleic acid. This compound is mainly found in low-density lipoprotein (LDL) particles and plays a crucial role in cholesterol transport and storage .
Mode of Action
this compound interacts with enzymes involved in cholesterol metabolism, particularly sterol O-acyltransferase (SOAT), also known as acyl-coenzyme A: cholesterol acyltransferase (ACAT). SOAT catalyzes the esterification of cholesterol, converting free cholesterol into cholesteryl esters like this compound. This process helps in the storage of cholesterol in lipid droplets within cells .
Biochemical Pathways
The primary biochemical pathway affected by this compound is the cholesterol esterification pathway. This pathway involves the conversion of free cholesterol into cholesteryl esters, which are then incorporated into lipoproteins for transport. The esterification process reduces the cytotoxicity of free cholesterol and facilitates its storage and transport. Downstream effects include the regulation of cholesterol homeostasis and prevention of cholesterol accumulation in cell membranes .
Pharmacokinetics
this compound’s pharmacokinetics involve its absorption, distribution, metabolism, and excretion (ADME). After dietary intake, this compound is absorbed in the intestines and incorporated into chylomicrons. It is then transported to the liver, where it is repackaged into very low-density lipoproteins (VLDL) and eventually converted into LDL particles. The bioavailability of this compound is influenced by factors such as dietary fat content and the presence of bile acids .
Result of Action
At the molecular level, this compound’s action results in the formation of lipid droplets within cells, aiding in cholesterol storage. At the cellular level, it helps maintain cholesterol homeostasis by preventing the accumulation of free cholesterol, which can be cytotoxic. This regulation is crucial for cellular functions and overall lipid metabolism .
Action Environment
Environmental factors such as diet, temperature, and pH can influence the action, efficacy, and stability of this compound. A diet high in fats can enhance the absorption and transport of this compound. Temperature and pH can affect the stability of this compound, with extreme conditions potentially leading to its degradation .
This compound plays a significant role in cholesterol metabolism, aiding in the storage and transport of cholesterol, and maintaining cellular cholesterol homeostasis. Understanding its mechanism of action provides insights into its potential therapeutic applications and its role in lipid metabolism disorders.
: MedChemExpress : Nature : BMC Pharmacology : Sigma-Aldrich : Wikipedia : MedChemExpress
Biochemical Analysis
Biochemical Properties
Cholesteryl oleate is involved in several biochemical reactions, primarily related to lipid metabolism. It interacts with enzymes such as lecithin-cholesterol acyltransferase (LCAT) and cholesterol esterase. LCAT catalyzes the formation of this compound from cholesterol and oleic acid, while cholesterol esterase hydrolyzes this compound back into cholesterol and oleic acid . These interactions are essential for maintaining cholesterol homeostasis and facilitating the transport of cholesterol in a more hydrophobic form within lipoprotein particles .
Cellular Effects
This compound has significant effects on various cell types and cellular processes. It is a major constituent of lipid droplets in cells, particularly in macrophages, where it contributes to the formation of foam cells, a hallmark of atherosclerosis . This compound influences cell signaling pathways, gene expression, and cellular metabolism by modulating the availability of cholesterol and fatty acids. It also affects the activity of enzymes involved in lipid metabolism, such as acyl-CoA:cholesterol acyltransferase (ACAT), which esterifies cholesterol to form this compound .
Molecular Mechanism
At the molecular level, this compound exerts its effects through various mechanisms. It binds to and is hydrolyzed by cholesterol esterase, releasing free cholesterol and oleic acid . This hydrolysis is crucial for the mobilization of cholesterol from lipid droplets and its subsequent utilization in cellular processes. This compound also interacts with lipid transport proteins, such as scavenger receptor class B type I (SR-BI), facilitating the uptake and efflux of cholesterol in cells . Additionally, it influences gene expression by modulating the activity of transcription factors involved in lipid metabolism .
Temporal Effects in Laboratory Settings
In laboratory settings, the effects of this compound can change over time. Studies have shown that this compound is relatively stable under physiological conditions but can undergo hydrolysis by cholesterol esterase over time . Long-term exposure to this compound in cell culture models has been associated with alterations in cellular lipid metabolism and the formation of lipid droplets . These changes can impact cellular function and viability, particularly in macrophages and other lipid-storing cells.
Dosage Effects in Animal Models
The effects of this compound vary with different dosages in animal models. At low doses, this compound is efficiently metabolized and incorporated into lipid droplets without causing significant toxicity . At high doses, this compound can lead to the accumulation of lipid droplets and the formation of foam cells, contributing to the development of atherosclerosis . Toxic or adverse effects at high doses include increased oxidative stress and inflammation in vascular tissues .
Metabolic Pathways
This compound is involved in several metabolic pathways, including the esterification and hydrolysis of cholesterol. It is synthesized by the action of LCAT, which transfers an acyl group from phosphatidylcholine to cholesterol, forming this compound . The hydrolysis of this compound is catalyzed by cholesterol esterase, releasing free cholesterol and oleic acid . These metabolic pathways are essential for maintaining cholesterol homeostasis and regulating lipid storage and transport within cells .
Transport and Distribution
This compound is transported and distributed within cells and tissues through lipoprotein particles, primarily LDL . It is taken up by cells via receptor-mediated endocytosis and incorporated into lipid droplets for storage . This compound can also be transported between lipoprotein particles by cholesteryl ester transfer protein (CETP), facilitating the redistribution of cholesterol within the body . The transport and distribution of this compound are critical for maintaining lipid balance and preventing the accumulation of cholesterol in tissues .
Subcellular Localization
This compound is primarily localized in lipid droplets within cells . These lipid droplets serve as storage sites for this compound and other neutral lipids. The subcellular localization of this compound is regulated by targeting signals and post-translational modifications that direct it to specific compartments or organelles . The presence of this compound in lipid droplets is essential for maintaining cellular lipid homeostasis and providing a reservoir of cholesterol for cellular processes .
Preparation Methods
Synthetic Routes and Reaction Conditions
Cholesteryl oleate can be synthesized through the esterification of cholesterol with oleic acid. One common method involves the use of cholesteryl acetate and methyl oleate, with sodium ethylate as a catalyst. The reaction is carried out under reflux conditions, typically in a benzene solution at temperatures ranging from 130°C to 140°C .
Industrial Production Methods
In industrial settings, this compound is often produced through the esterification of cholesterol with free fatty acids, acid anhydrides, or acid chlorides. The process may involve heating cholesterol and the appropriate fatty acid at high temperatures (around 200°C) in an atmosphere of carbon dioxide. Acid catalysts are also used to facilitate the reaction, although this method may result in lower yields and the formation of side products .
Chemical Reactions Analysis
Types of Reactions
Cholesteryl oleate undergoes various chemical reactions, including:
Oxidation: this compound can be oxidized to form cholesteryl ester hydroperoxides, which are intermediates in the formation of atherosclerotic plaques.
Transesterification: this compound can undergo transesterification reactions with other fatty acids, leading to the formation of different cholesteryl esters.
Common Reagents and Conditions
Oxidation: Common oxidizing agents include hydrogen peroxide and various peroxides.
Hydrolysis: Enzymatic hydrolysis is typically carried out using cholesterol esterase under physiological conditions.
Transesterification: Sodium ethylate or other alkoxide catalysts are used in transesterification reactions.
Major Products Formed
Oxidation: Cholesteryl ester hydroperoxides.
Hydrolysis: Cholesterol and oleic acid.
Transesterification: Different cholesteryl esters depending on the fatty acid used.
Scientific Research Applications
Comparison with Similar Compounds
Similar Compounds
Cholesteryl linoleate: Another cholesteryl ester formed from cholesterol and linoleic acid.
Cholesteryl palmitate: Formed from cholesterol and palmitic acid.
Cholesteryl stearate: Formed from cholesterol and stearic acid.
Uniqueness
Cholesteryl oleate is unique due to its specific fatty acid component, oleic acid, which is a monounsaturated fatty acid. This gives this compound distinct physical and chemical properties compared to other cholesteryl esters. For example, this compound has a lower melting point and different solubility characteristics, which can influence its behavior in biological systems .
Properties
IUPAC Name |
[10,13-dimethyl-17-(6-methylheptan-2-yl)-2,3,4,7,8,9,11,12,14,15,16,17-dodecahydro-1H-cyclopenta[a]phenanthren-3-yl] octadec-9-enoate | |
---|---|---|
Details | Computed by Lexichem TK 2.7.0 (PubChem release 2021.05.07) | |
Source | PubChem | |
URL | https://pubchem.ncbi.nlm.nih.gov | |
Description | Data deposited in or computed by PubChem | |
InChI |
InChI=1S/C45H78O2/c1-7-8-9-10-11-12-13-14-15-16-17-18-19-20-21-25-43(46)47-38-30-32-44(5)37(34-38)26-27-39-41-29-28-40(36(4)24-22-23-35(2)3)45(41,6)33-31-42(39)44/h14-15,26,35-36,38-42H,7-13,16-25,27-34H2,1-6H3 | |
Details | Computed by InChI 1.0.6 (PubChem release 2021.05.07) | |
Source | PubChem | |
URL | https://pubchem.ncbi.nlm.nih.gov | |
Description | Data deposited in or computed by PubChem | |
InChI Key |
RJECHNNFRHZQKU-UHFFFAOYSA-N | |
Details | Computed by InChI 1.0.6 (PubChem release 2021.05.07) | |
Source | PubChem | |
URL | https://pubchem.ncbi.nlm.nih.gov | |
Description | Data deposited in or computed by PubChem | |
Canonical SMILES |
CCCCCCCCC=CCCCCCCCC(=O)OC1CCC2(C3CCC4(C(C3CC=C2C1)CCC4C(C)CCCC(C)C)C)C | |
Details | Computed by OEChem 2.3.0 (PubChem release 2021.05.07) | |
Source | PubChem | |
URL | https://pubchem.ncbi.nlm.nih.gov | |
Description | Data deposited in or computed by PubChem | |
Molecular Formula |
C45H78O2 | |
Details | Computed by PubChem 2.1 (PubChem release 2021.05.07) | |
Source | PubChem | |
URL | https://pubchem.ncbi.nlm.nih.gov | |
Description | Data deposited in or computed by PubChem | |
Molecular Weight |
651.1 g/mol | |
Details | Computed by PubChem 2.1 (PubChem release 2021.05.07) | |
Source | PubChem | |
URL | https://pubchem.ncbi.nlm.nih.gov | |
Description | Data deposited in or computed by PubChem | |
Retrosynthesis Analysis
AI-Powered Synthesis Planning: Our tool employs the Template_relevance Pistachio, Template_relevance Bkms_metabolic, Template_relevance Pistachio_ringbreaker, Template_relevance Reaxys, Template_relevance Reaxys_biocatalysis model, leveraging a vast database of chemical reactions to predict feasible synthetic routes.
One-Step Synthesis Focus: Specifically designed for one-step synthesis, it provides concise and direct routes for your target compounds, streamlining the synthesis process.
Accurate Predictions: Utilizing the extensive PISTACHIO, BKMS_METABOLIC, PISTACHIO_RINGBREAKER, REAXYS, REAXYS_BIOCATALYSIS database, our tool offers high-accuracy predictions, reflecting the latest in chemical research and data.
Strategy Settings
Precursor scoring | Relevance Heuristic |
---|---|
Min. plausibility | 0.01 |
Model | Template_relevance |
Template Set | Pistachio/Bkms_metabolic/Pistachio_ringbreaker/Reaxys/Reaxys_biocatalysis |
Top-N result to add to graph | 6 |
Feasible Synthetic Routes
Disclaimer and Information on In-Vitro Research Products
Please be aware that all articles and product information presented on BenchChem are intended solely for informational purposes. The products available for purchase on BenchChem are specifically designed for in-vitro studies, which are conducted outside of living organisms. In-vitro studies, derived from the Latin term "in glass," involve experiments performed in controlled laboratory settings using cells or tissues. It is important to note that these products are not categorized as medicines or drugs, and they have not received approval from the FDA for the prevention, treatment, or cure of any medical condition, ailment, or disease. We must emphasize that any form of bodily introduction of these products into humans or animals is strictly prohibited by law. It is essential to adhere to these guidelines to ensure compliance with legal and ethical standards in research and experimentation.