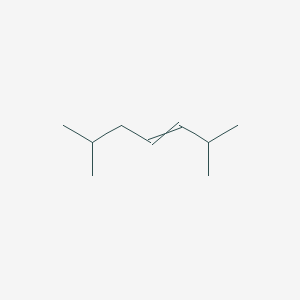
2,6-Dimethylhept-3-ene
Overview
Description
2,6-Dimethylhept-3-ene is a chemical compound with the molecular formula C9H18 . It has a molecular weight of 126.239 Da . The compound is also known by other names such as 3-Heptene, 2,6-dimethyl-, and its CAS Registry Number is 2738-18-3 .
Molecular Structure Analysis
The molecular structure of 2,6-Dimethylhept-3-ene can be viewed as a 2D Mol file or as a computed 3D SD file . The structure is based on structures generated from information available in databases .Physical And Chemical Properties Analysis
The physical and chemical properties of 2,6-Dimethylhept-3-ene include a molecular formula of CH, an average mass of 126.239 Da, and a monoisotopic mass of 126.140854 Da .Scientific Research Applications
Chemical Vapor Deposition (CVD)
These applications highlight the compound’s versatility and importance across various scientific domains. If you need further details or additional applications, feel free to ask! 🌟
Safety And Hazards
properties
IUPAC Name |
2,6-dimethylhept-3-ene | |
---|---|---|
Source | PubChem | |
URL | https://pubchem.ncbi.nlm.nih.gov | |
Description | Data deposited in or computed by PubChem | |
InChI |
InChI=1S/C9H18/c1-8(2)6-5-7-9(3)4/h5-6,8-9H,7H2,1-4H3 | |
Source | PubChem | |
URL | https://pubchem.ncbi.nlm.nih.gov | |
Description | Data deposited in or computed by PubChem | |
InChI Key |
KDISTZUHDQPXDE-UHFFFAOYSA-N | |
Source | PubChem | |
URL | https://pubchem.ncbi.nlm.nih.gov | |
Description | Data deposited in or computed by PubChem | |
Canonical SMILES |
CC(C)CC=CC(C)C | |
Source | PubChem | |
URL | https://pubchem.ncbi.nlm.nih.gov | |
Description | Data deposited in or computed by PubChem | |
Molecular Formula |
C9H18 | |
Source | PubChem | |
URL | https://pubchem.ncbi.nlm.nih.gov | |
Description | Data deposited in or computed by PubChem | |
DSSTOX Substance ID |
DTXSID101314150 | |
Record name | 2,6-Dimethyl-3-heptene | |
Source | EPA DSSTox | |
URL | https://comptox.epa.gov/dashboard/DTXSID101314150 | |
Description | DSSTox provides a high quality public chemistry resource for supporting improved predictive toxicology. | |
Molecular Weight |
126.24 g/mol | |
Source | PubChem | |
URL | https://pubchem.ncbi.nlm.nih.gov | |
Description | Data deposited in or computed by PubChem | |
Product Name |
2,6-Dimethylhept-3-ene | |
CAS RN |
2738-18-3 | |
Record name | 2,6-Dimethyl-3-heptene | |
Source | CAS Common Chemistry | |
URL | https://commonchemistry.cas.org/detail?cas_rn=2738-18-3 | |
Description | CAS Common Chemistry is an open community resource for accessing chemical information. Nearly 500,000 chemical substances from CAS REGISTRY cover areas of community interest, including common and frequently regulated chemicals, and those relevant to high school and undergraduate chemistry classes. This chemical information, curated by our expert scientists, is provided in alignment with our mission as a division of the American Chemical Society. | |
Explanation | The data from CAS Common Chemistry is provided under a CC-BY-NC 4.0 license, unless otherwise stated. | |
Record name | 2,6-Dimethyl-3-heptene | |
Source | EPA DSSTox | |
URL | https://comptox.epa.gov/dashboard/DTXSID101314150 | |
Description | DSSTox provides a high quality public chemistry resource for supporting improved predictive toxicology. | |
Record name | 2,6-dimethylhept-3-ene | |
Source | European Chemicals Agency (ECHA) | |
URL | https://echa.europa.eu/substance-information/-/substanceinfo/100.018.511 | |
Description | The European Chemicals Agency (ECHA) is an agency of the European Union which is the driving force among regulatory authorities in implementing the EU's groundbreaking chemicals legislation for the benefit of human health and the environment as well as for innovation and competitiveness. | |
Explanation | Use of the information, documents and data from the ECHA website is subject to the terms and conditions of this Legal Notice, and subject to other binding limitations provided for under applicable law, the information, documents and data made available on the ECHA website may be reproduced, distributed and/or used, totally or in part, for non-commercial purposes provided that ECHA is acknowledged as the source: "Source: European Chemicals Agency, http://echa.europa.eu/". Such acknowledgement must be included in each copy of the material. ECHA permits and encourages organisations and individuals to create links to the ECHA website under the following cumulative conditions: Links can only be made to webpages that provide a link to the Legal Notice page. | |
Retrosynthesis Analysis
AI-Powered Synthesis Planning: Our tool employs the Template_relevance Pistachio, Template_relevance Bkms_metabolic, Template_relevance Pistachio_ringbreaker, Template_relevance Reaxys, Template_relevance Reaxys_biocatalysis model, leveraging a vast database of chemical reactions to predict feasible synthetic routes.
One-Step Synthesis Focus: Specifically designed for one-step synthesis, it provides concise and direct routes for your target compounds, streamlining the synthesis process.
Accurate Predictions: Utilizing the extensive PISTACHIO, BKMS_METABOLIC, PISTACHIO_RINGBREAKER, REAXYS, REAXYS_BIOCATALYSIS database, our tool offers high-accuracy predictions, reflecting the latest in chemical research and data.
Strategy Settings
Precursor scoring | Relevance Heuristic |
---|---|
Min. plausibility | 0.01 |
Model | Template_relevance |
Template Set | Pistachio/Bkms_metabolic/Pistachio_ringbreaker/Reaxys/Reaxys_biocatalysis |
Top-N result to add to graph | 6 |
Feasible Synthetic Routes
Q & A
Q1: How does the structure of 2,6-Dimethylhept-3-ene influence its reactivity with ozone?
A1: 2,6-Dimethylhept-3-ene possesses a simple alkene functional group, which is known to react readily with ozone. Interestingly, research indicates that the presence of specific substituents near the double bond can significantly alter the reaction outcome. For instance, while 4-alkylthio-2,6-dimethylhept-3-ene undergoes the expected double bond cleavage with ozone [], certain derivatives of thiopinacolone and thiocamphor containing the 2,6-dimethylhept-3-ene backbone yield products where the hydrocarbon chain remains intact []. This suggests that steric hindrance or electronic effects imposed by bulky substituents near the double bond can influence the reaction pathway. Further research is needed to fully elucidate the factors governing this intriguing reactivity pattern.
Disclaimer and Information on In-Vitro Research Products
Please be aware that all articles and product information presented on BenchChem are intended solely for informational purposes. The products available for purchase on BenchChem are specifically designed for in-vitro studies, which are conducted outside of living organisms. In-vitro studies, derived from the Latin term "in glass," involve experiments performed in controlled laboratory settings using cells or tissues. It is important to note that these products are not categorized as medicines or drugs, and they have not received approval from the FDA for the prevention, treatment, or cure of any medical condition, ailment, or disease. We must emphasize that any form of bodily introduction of these products into humans or animals is strictly prohibited by law. It is essential to adhere to these guidelines to ensure compliance with legal and ethical standards in research and experimentation.