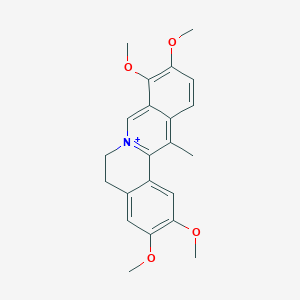
Dehydrocorydaline
Overview
Description
Dehydrocorydaline is a quaternary alkaloid compound isolated from the traditional Chinese herb Corydalis yanhusuo . It has been demonstrated to possess various pharmacological properties, including anti-inflammatory, antithrombotic, and cardioprotective effects . This compound has garnered significant attention in scientific research due to its potential therapeutic applications.
Mechanism of Action
Target of Action
Dehydrocorydaline, an alkaloidal compound isolated from the traditional Chinese herb Corydalis yanhusuo , has been found to target multiple sites in the body. It primarily targets macrophages , and it has been shown to combat Listeria monocytogenes by dysregulating carbohydrate metabolism, suppressing cell wall synthesis, and inhibiting bacterial motility . It also targets the TRAF6/NF-κB pathway and the MEK1/2-ERK1/2 cascade .
Mode of Action
This compound interacts with its targets in a variety of ways. It has been shown to decrease the levels of proinflammatory gene clusters, significantly downregulate proinflammatory interleukin (IL)-1β and IL-18 mRNA levels in a time- and concentration-dependent manner . Furthermore, it decreases lipopolysaccharide (LPS)-induced inflammation in bone marrow-derived macrophages (BMDMs), as evidenced by the reduced protein levels of CD80, iNOS, NLRP3, IL-1β, and IL-18 . Importantly, it attenuates LPS-induced activation of p65 and the extracellular signal-regulated kinase 1/2 (ERK1/2) pathway .
Biochemical Pathways
This compound affects several biochemical pathways. It inhibits the TRAF6/NF-κB pathway , which plays a crucial role in immune and inflammatory responses. It also suppresses the MEK1/2-ERK1/2 cascade , which is involved in regulating cell proliferation, migration, and invasion. In addition, it has been shown to dysregulate carbohydrate metabolism, suppress cell wall synthesis, and inhibit bacterial motility in Listeria monocytogenes .
Pharmacokinetics
It’s known that it’s an alkaloidal compound isolated from the traditional chinese herb corydalis yanhusuo , suggesting it’s likely to be absorbed and metabolized in the body
Result of Action
This compound has been shown to have significant anti-inflammatory and anti-oxidative stress functions . It decreases IL-1β, IL-6, TNFα, and IFNγ levels, mitigates ROS level, and up-regulates Nrf2/HO-1, SOD, and GSH-PX expressions dose-dependently . It also improves the survival of sepsis mice, reduces TUNEL-labeled apoptosis of cardiomyocytes, and enhances the viability of LPS-induced H9C2 cardiomyocytes .
Action Environment
The action of this compound can be influenced by environmental factors. For instance, it has been shown to have anti-inflammatory and antithrombotic benefits , suggesting that it may be particularly effective in environments where inflammation and thrombosis are prevalent.
Biochemical Analysis
Biochemical Properties
Dehydrocorydaline interacts with various enzymes and proteins, playing a significant role in biochemical reactions. It has been found to inhibit the TRAF6/NF-κB pathway, which is crucial in inflammatory responses . This compound also interacts with enzymes involved in the production of inflammatory cytokines, such as IL-1β, IL-6, and TNFα .
Cellular Effects
This compound has notable effects on various types of cells and cellular processes. It has been shown to protect against sepsis-induced myocardial injury by enhancing the viability of LPS-induced H9C2 cardiomyocytes and inhibiting cell apoptosis . This compound also influences cell function by modulating cell signaling pathways, gene expression, and cellular metabolism .
Molecular Mechanism
This compound exerts its effects at the molecular level through several mechanisms. It inhibits the TRAF6 expression and the phosphorylation of NF-κB p65, which are key components of the inflammatory response . This compound also impacts gene expression, leading to decreased levels of proinflammatory cytokines .
Temporal Effects in Laboratory Settings
Over time, this compound has shown to have stable and long-term effects on cellular function in both in vitro and in vivo studies . It has been observed to consistently inhibit inflammation and oxidative stress in sepsis-induced cardiomyocyte injury models .
Dosage Effects in Animal Models
In animal models, the effects of this compound vary with different dosages. At higher doses, it has shown significant antinociceptive effects in mouse models of inflammatory pain
Metabolic Pathways
This compound is involved in several metabolic pathways. It has been found to influence carbohydrate metabolism, cell wall synthesis, and bacterial motility . It interacts with enzymes and cofactors, potentially affecting metabolic flux or metabolite levels .
Transport and Distribution
This compound is transported and distributed within cells and tissues. It has been reported that Organic Cation Transporter 1 and 3 (OCT1/3) contribute to the high accumulation of this compound in the heart .
Preparation Methods
Synthetic Routes and Reaction Conditions: Dehydrocorydaline can be synthesized through various chemical reactions involving the precursor compounds found in Corydalis yanhusuo. The synthetic routes typically involve multiple steps, including methylation, demethylation, and oxidation reactions .
Industrial Production Methods: The industrial production of this compound involves the extraction and purification of the compound from Corydalis yanhusuo. The process includes the use of solvents such as methanol and ethanol for extraction, followed by chromatographic techniques for purification .
Chemical Reactions Analysis
Types of Reactions: Dehydrocorydaline undergoes several types of chemical reactions, including:
Oxidation: This reaction involves the addition of oxygen or the removal of hydrogen from the compound.
Reduction: This reaction involves the addition of hydrogen or the removal of oxygen from the compound.
Substitution: This reaction involves the replacement of one functional group with another.
Common Reagents and Conditions:
Oxidation: Common reagents include potassium permanganate and hydrogen peroxide.
Reduction: Common reagents include sodium borohydride and lithium aluminum hydride.
Substitution: Common reagents include halogens and nucleophiles.
Major Products Formed: The major products formed from these reactions include various derivatives of this compound, which may possess different pharmacological properties .
Scientific Research Applications
Comparison with Similar Compounds
- Berberine
- Palmatine
- Coptisine
Dehydrocorydaline’s unique pharmacological profile and multi-targeted mechanism of action distinguish it from other similar compounds, highlighting its potential as a versatile therapeutic agent.
Biological Activity
Dehydrocorydaline (DHC) is an alkaloid derived from the rhizome of Corydalis turschaninovii, which has garnered attention for its diverse biological activities. This article explores the antibacterial, anti-inflammatory, antinociceptive, and neuroprotective effects of DHC, supported by recent research findings and case studies.
1. Antibacterial Activity
DHC has been identified as a potent antibacterial agent, particularly against Listeria monocytogenes, a significant foodborne pathogen.
- Mechanism of Action : A study utilized high-performance liquid chromatography coupled with hybrid linear ion trap quadrupole-orbitrap mass spectrometry (HPLC-LTQ-Orbitrap-MS/MS) to analyze DHC's effects. It demonstrated that DHC exhibits a minimum inhibitory concentration (MIC) of 1 mg/mL and a minimum bactericidal concentration (MBC) of 2 mg/mL against L. monocytogenes .
- Multi-target Mechanisms : Proteomic analysis revealed that DHC disrupts carbohydrate metabolism, inhibits cell wall synthesis, and impairs bacterial motility, indicating its multi-target action against bacterial cells .
Parameter | Value |
---|---|
Minimum Inhibitory Concentration (MIC) | 1 mg/mL |
Minimum Bactericidal Concentration (MBC) | 2 mg/mL |
2. Anti-inflammatory and Antinociceptive Effects
DHC exhibits significant anti-inflammatory properties and has been shown to alleviate pain in various models.
- Antinociceptive Effects : Research involving acetic acid-induced writhing tests and formalin paw tests in mice demonstrated that DHC provided a dose-dependent reduction in pain responses without affecting locomotor activity. The effective doses ranged from 3.6 to 10 mg/kg .
- Inflammatory Mediators : DHC administration led to decreased levels of pro-inflammatory cytokines such as TNF-α, IL-1β, and IL-6 in the spinal cord, suggesting its potential in managing inflammatory pain .
3. Neuroprotective Effects
DHC has shown promise in neuroprotection, particularly in models of depression.
- Chronic Unpredictable Mild Stress (CUMS) : In CUMS mouse models, DHC treatment significantly improved depressive-like behaviors and reduced neuronal damage in the hippocampus. Behavioral assays indicated increased sucrose preference and reduced immobility times in treated groups compared to controls .
- Mechanistic Insights : The neuroprotective effects are linked to the modulation of astrocyte activation via the NLRP3 inflammasome pathway, which is crucial for neuroinflammation and neuronal survival .
4. Cardiovascular Protection
Emerging studies suggest that DHC may also protect cardiovascular health.
- Atherosclerosis Model : In apolipoprotein E-deficient (ApoE−/−) mice, DHC treatment reduced atherosclerotic lesions by inhibiting macrophage-mediated inflammation through suppression of ERK1/2 signaling pathways .
Case Studies
Several studies highlight the varied applications of DHC:
- Breast Cancer Inhibition : DHC has been shown to inhibit the proliferation of MCF-7 breast cancer cells in vitro, indicating its potential as an anti-cancer agent .
- Bone Cancer Pain Management : Research indicates that DHC can attenuate bone cancer pain by shifting microglial polarization states in the spinal cord .
Properties
IUPAC Name |
2,3,9,10-tetramethoxy-13-methyl-5,6-dihydroisoquinolino[2,1-b]isoquinolin-7-ium | |
---|---|---|
Source | PubChem | |
URL | https://pubchem.ncbi.nlm.nih.gov | |
Description | Data deposited in or computed by PubChem | |
InChI |
InChI=1S/C22H24NO4/c1-13-15-6-7-18(24-2)22(27-5)17(15)12-23-9-8-14-10-19(25-3)20(26-4)11-16(14)21(13)23/h6-7,10-12H,8-9H2,1-5H3/q+1 | |
Source | PubChem | |
URL | https://pubchem.ncbi.nlm.nih.gov | |
Description | Data deposited in or computed by PubChem | |
InChI Key |
RFKQJTRWODZPHF-UHFFFAOYSA-N | |
Source | PubChem | |
URL | https://pubchem.ncbi.nlm.nih.gov | |
Description | Data deposited in or computed by PubChem | |
Canonical SMILES |
CC1=C2C=CC(=C(C2=C[N+]3=C1C4=CC(=C(C=C4CC3)OC)OC)OC)OC | |
Source | PubChem | |
URL | https://pubchem.ncbi.nlm.nih.gov | |
Description | Data deposited in or computed by PubChem | |
Molecular Formula |
C22H24NO4+ | |
Source | PubChem | |
URL | https://pubchem.ncbi.nlm.nih.gov | |
Description | Data deposited in or computed by PubChem | |
Related CAS |
10605-03-5 (chloride) | |
Record name | Dehydrocorydalin | |
Source | ChemIDplus | |
URL | https://pubchem.ncbi.nlm.nih.gov/substance/?source=chemidplus&sourceid=0030045160 | |
Description | ChemIDplus is a free, web search system that provides access to the structure and nomenclature authority files used for the identification of chemical substances cited in National Library of Medicine (NLM) databases, including the TOXNET system. | |
DSSTOX Substance ID |
DTXSID30904183 | |
Record name | Dehydrocorydalin | |
Source | EPA DSSTox | |
URL | https://comptox.epa.gov/dashboard/DTXSID30904183 | |
Description | DSSTox provides a high quality public chemistry resource for supporting improved predictive toxicology. | |
Molecular Weight |
366.4 g/mol | |
Source | PubChem | |
URL | https://pubchem.ncbi.nlm.nih.gov | |
Description | Data deposited in or computed by PubChem | |
CAS No. |
30045-16-0 | |
Record name | Dehydrocorydaline | |
Source | CAS Common Chemistry | |
URL | https://commonchemistry.cas.org/detail?cas_rn=30045-16-0 | |
Description | CAS Common Chemistry is an open community resource for accessing chemical information. Nearly 500,000 chemical substances from CAS REGISTRY cover areas of community interest, including common and frequently regulated chemicals, and those relevant to high school and undergraduate chemistry classes. This chemical information, curated by our expert scientists, is provided in alignment with our mission as a division of the American Chemical Society. | |
Explanation | The data from CAS Common Chemistry is provided under a CC-BY-NC 4.0 license, unless otherwise stated. | |
Record name | Dehydrocorydalin | |
Source | ChemIDplus | |
URL | https://pubchem.ncbi.nlm.nih.gov/substance/?source=chemidplus&sourceid=0030045160 | |
Description | ChemIDplus is a free, web search system that provides access to the structure and nomenclature authority files used for the identification of chemical substances cited in National Library of Medicine (NLM) databases, including the TOXNET system. | |
Record name | Dehydrocorydalin | |
Source | EPA DSSTox | |
URL | https://comptox.epa.gov/dashboard/DTXSID30904183 | |
Description | DSSTox provides a high quality public chemistry resource for supporting improved predictive toxicology. | |
Retrosynthesis Analysis
AI-Powered Synthesis Planning: Our tool employs the Template_relevance Pistachio, Template_relevance Bkms_metabolic, Template_relevance Pistachio_ringbreaker, Template_relevance Reaxys, Template_relevance Reaxys_biocatalysis model, leveraging a vast database of chemical reactions to predict feasible synthetic routes.
One-Step Synthesis Focus: Specifically designed for one-step synthesis, it provides concise and direct routes for your target compounds, streamlining the synthesis process.
Accurate Predictions: Utilizing the extensive PISTACHIO, BKMS_METABOLIC, PISTACHIO_RINGBREAKER, REAXYS, REAXYS_BIOCATALYSIS database, our tool offers high-accuracy predictions, reflecting the latest in chemical research and data.
Strategy Settings
Precursor scoring | Relevance Heuristic |
---|---|
Min. plausibility | 0.01 |
Model | Template_relevance |
Template Set | Pistachio/Bkms_metabolic/Pistachio_ringbreaker/Reaxys/Reaxys_biocatalysis |
Top-N result to add to graph | 6 |
Feasible Synthetic Routes
Q1: How does Dehydrocorydaline exert its anti-metastatic effects in Non-Small Cell Lung Carcinoma (NSCLC) cells?
A1: this compound demonstrates anti-metastatic activity in NSCLC cells through a multifaceted mechanism. Primarily, it targets the B-cell lymphoma 2 (Bcl-2) signaling pathway, downregulating the expression of the anti-apoptotic protein Bcl-2 while promoting the expression of the pro-apoptotic protein Bax []. Additionally, this compound effectively suppresses the expression of matrix metalloproteinases (MMPs), specifically MMP-7 and MMP-9, which are key enzymes involved in the breakdown of the extracellular matrix, facilitating cancer cell invasion and metastasis [].
Q2: Are there any studies investigating the impact of this compound on specific molecular targets within the Bcl-2 signaling pathway?
A2: While the provided research highlights the interaction of this compound with the Bcl-2 signaling pathway, further investigation is needed to elucidate the precise molecular targets within this pathway. Future studies focusing on identifying specific interactions between this compound and key proteins within the Bcl-2 pathway will be crucial for a comprehensive understanding of its anti-tumor mechanisms.
Q3: What is the historical context of this compound research?
A3: Early research on this compound, specifically the study "Ueber das r‐Corydalin" [], primarily focused on its structural characterization and synthesis. This laid the groundwork for subsequent investigations exploring its biological activities. More recently, studies like the one highlighting its anti-metastatic effects in NSCLC cells [] demonstrate a shift towards understanding its potential therapeutic applications.
Disclaimer and Information on In-Vitro Research Products
Please be aware that all articles and product information presented on BenchChem are intended solely for informational purposes. The products available for purchase on BenchChem are specifically designed for in-vitro studies, which are conducted outside of living organisms. In-vitro studies, derived from the Latin term "in glass," involve experiments performed in controlled laboratory settings using cells or tissues. It is important to note that these products are not categorized as medicines or drugs, and they have not received approval from the FDA for the prevention, treatment, or cure of any medical condition, ailment, or disease. We must emphasize that any form of bodily introduction of these products into humans or animals is strictly prohibited by law. It is essential to adhere to these guidelines to ensure compliance with legal and ethical standards in research and experimentation.