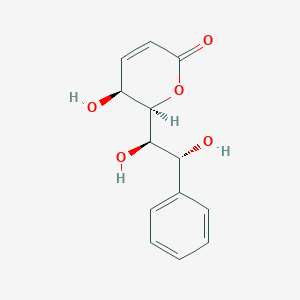
Goniotriol
Overview
Description
Goniotriol is a styrylpyrone that can be found in Goniothalamus amuyon . It shows cytotoxicity and is a natural phenol found in the herbs of Goniothalamus giganteus .
Synthesis Analysis
The synthesis of Goniotriol involves several steps, including selective deacetonation, glycol cleavage oxidation, and Wittig reaction . More detailed information about the synthesis process can be found in the referenced papers .Molecular Structure Analysis
The molecular formula of Goniotriol is C13H14O5 . Its molecular weight is 250.25 . More detailed structural analysis can be found in the referenced papers .Scientific Research Applications
Cytotoxic Agent in Cancer Research
Goniotriol has been identified as a compound with cytotoxic properties . It is found in the plant Goniothalamus amuyon and has shown potential in cancer research for its ability to induce cell death in various cancer cell lines. Studies have reported its effectiveness against cell lines such as KB, P-388, A-549, HT-29, and HL-60, with varying degrees of potency .
Pharmacological Studies
In pharmacology, Goniotriol’s cytotoxicity suggests a potential for developing anti-cancer therapies. Its mechanism of action and the pathways it affects are areas of ongoing research, which could lead to new insights into cancer treatment strategies .
Chemical Synthesis and Modification
Goniotriol serves as a base chemical structure for synthetic modifications. Researchers aim to enhance its pharmacological properties or reduce toxicity by altering its chemical structure, leading to the synthesis of analogs with potentially improved therapeutic profiles .
Biotechnology Applications
The cytotoxic nature of Goniotriol makes it a candidate for biotechnological applications, particularly in the development of bioassays for screening other compounds with cytotoxic or anti-cancer properties .
Environmental Applications
While direct references to Goniotriol’s environmental applications are limited, the study of its cytotoxic effects can contribute to environmental risk assessments, particularly in evaluating the impact of bioactive compounds on ecosystems .
Material Science
In material science, the chemical properties of Goniotriol could be studied for the development of new materials, such as bioactive coatings or components in nanotechnology, which could have medical or industrial applications .
Agricultural Research
Goniotriol’s bioactivity may also be explored in agricultural research, particularly in the development of natural pesticides or growth inhibitors, given its cytotoxic effects on certain cell lines .
Pharmacokinetics and Drug Delivery
Understanding the pharmacokinetics of Goniotriol is crucial for its potential therapeutic use. Research in this area could lead to the development of novel drug delivery systems that optimize its bioavailability and therapeutic efficacy .
Mechanism of Action
Target of Action
Goniotriol, a styrylpyrone found in Goniothalamus amuyon , primarily targets cancer cells . It exhibits cytotoxicity, making it a potential candidate for cancer treatment .
Mode of Action
Goniotriol interacts with its targets (cancer cells) by inducing cytotoxicity .
Biochemical Pathways
It’s known that goniotriol exhibits cytotoxicity, suggesting that it may interfere with cell proliferation and survival pathways
Result of Action
Goniotriol shows cytotoxicity with ED 50 s of 6.23, 1.38, 4.12, 4.89, 2.31μg/mL for KB, P-388, A-549, HT-29, HL-60 cells, respectively . This suggests that Goniotriol can induce cell death in these cancer cell lines, thereby inhibiting their proliferation.
Safety and Hazards
properties
IUPAC Name |
(2R,3S)-2-[(1R,2R)-1,2-dihydroxy-2-phenylethyl]-3-hydroxy-2,3-dihydropyran-6-one | |
---|---|---|
Source | PubChem | |
URL | https://pubchem.ncbi.nlm.nih.gov | |
Description | Data deposited in or computed by PubChem | |
InChI |
InChI=1S/C13H14O5/c14-9-6-7-10(15)18-13(9)12(17)11(16)8-4-2-1-3-5-8/h1-7,9,11-14,16-17H/t9-,11+,12+,13+/m0/s1 | |
Source | PubChem | |
URL | https://pubchem.ncbi.nlm.nih.gov | |
Description | Data deposited in or computed by PubChem | |
InChI Key |
AWCDBKHWVKLXEE-WKSBVSIWSA-N | |
Source | PubChem | |
URL | https://pubchem.ncbi.nlm.nih.gov | |
Description | Data deposited in or computed by PubChem | |
Canonical SMILES |
C1=CC=C(C=C1)C(C(C2C(C=CC(=O)O2)O)O)O | |
Source | PubChem | |
URL | https://pubchem.ncbi.nlm.nih.gov | |
Description | Data deposited in or computed by PubChem | |
Isomeric SMILES |
C1=CC=C(C=C1)[C@H]([C@H]([C@H]2[C@H](C=CC(=O)O2)O)O)O | |
Source | PubChem | |
URL | https://pubchem.ncbi.nlm.nih.gov | |
Description | Data deposited in or computed by PubChem | |
Molecular Formula |
C13H14O5 | |
Source | PubChem | |
URL | https://pubchem.ncbi.nlm.nih.gov | |
Description | Data deposited in or computed by PubChem | |
DSSTOX Substance ID |
DTXSID501317251 | |
Record name | (+)-Goniotriol | |
Source | EPA DSSTox | |
URL | https://comptox.epa.gov/dashboard/DTXSID501317251 | |
Description | DSSTox provides a high quality public chemistry resource for supporting improved predictive toxicology. | |
Molecular Weight |
250.25 g/mol | |
Source | PubChem | |
URL | https://pubchem.ncbi.nlm.nih.gov | |
Description | Data deposited in or computed by PubChem | |
Product Name |
Goniotriol | |
CAS RN |
96405-62-8 | |
Record name | (+)-Goniotriol | |
Source | CAS Common Chemistry | |
URL | https://commonchemistry.cas.org/detail?cas_rn=96405-62-8 | |
Description | CAS Common Chemistry is an open community resource for accessing chemical information. Nearly 500,000 chemical substances from CAS REGISTRY cover areas of community interest, including common and frequently regulated chemicals, and those relevant to high school and undergraduate chemistry classes. This chemical information, curated by our expert scientists, is provided in alignment with our mission as a division of the American Chemical Society. | |
Explanation | The data from CAS Common Chemistry is provided under a CC-BY-NC 4.0 license, unless otherwise stated. | |
Record name | Goniotriol | |
Source | ChemIDplus | |
URL | https://pubchem.ncbi.nlm.nih.gov/substance/?source=chemidplus&sourceid=0096405628 | |
Description | ChemIDplus is a free, web search system that provides access to the structure and nomenclature authority files used for the identification of chemical substances cited in National Library of Medicine (NLM) databases, including the TOXNET system. | |
Record name | (+)-Goniotriol | |
Source | EPA DSSTox | |
URL | https://comptox.epa.gov/dashboard/DTXSID501317251 | |
Description | DSSTox provides a high quality public chemistry resource for supporting improved predictive toxicology. | |
Retrosynthesis Analysis
AI-Powered Synthesis Planning: Our tool employs the Template_relevance Pistachio, Template_relevance Bkms_metabolic, Template_relevance Pistachio_ringbreaker, Template_relevance Reaxys, Template_relevance Reaxys_biocatalysis model, leveraging a vast database of chemical reactions to predict feasible synthetic routes.
One-Step Synthesis Focus: Specifically designed for one-step synthesis, it provides concise and direct routes for your target compounds, streamlining the synthesis process.
Accurate Predictions: Utilizing the extensive PISTACHIO, BKMS_METABOLIC, PISTACHIO_RINGBREAKER, REAXYS, REAXYS_BIOCATALYSIS database, our tool offers high-accuracy predictions, reflecting the latest in chemical research and data.
Strategy Settings
Precursor scoring | Relevance Heuristic |
---|---|
Min. plausibility | 0.01 |
Model | Template_relevance |
Template Set | Pistachio/Bkms_metabolic/Pistachio_ringbreaker/Reaxys/Reaxys_biocatalysis |
Top-N result to add to graph | 6 |
Feasible Synthetic Routes
Q & A
Q1: What is the molecular formula and weight of goniotriol?
A1: Goniotriol possesses the molecular formula C14H14O5 and a molecular weight of 262.26 g/mol. [, , ]
Q2: What spectroscopic data is available for characterizing goniotriol?
A2: Researchers frequently employ Infrared (IR) spectroscopy, 1H Nuclear Magnetic Resonance (1H-NMR) spectroscopy, and Mass Spectrometry (MS) for the structural elucidation and confirmation of goniotriol. [, ] X-ray crystallography has also been used to determine the structure and relative stereochemistry of goniotriol. []
Q3: Are there any characteristic spectral features of goniotriol?
A3: Yes, the proton NMR spectra of substituted 5,7-O-isopropylidene-goniotriols and their C-7 epimers exhibit distinct chemical shifts for protons at the C5 position, providing a valuable tool for their differentiation. []
Q4: What are the primary biological activities reported for goniotriol?
A4: Goniotriol has demonstrated notable in vitro antitumor activity against various cancer cell lines, including A2780, HCT-8, KB, Bel742, and K562. [, , , ] Additionally, goniotriol exhibits antibacterial activity. []
Q5: What is the mechanism of action for goniotriol's antitumor activity?
A5: While the precise mechanism is yet to be fully elucidated, studies indicate that goniotriol can induce apoptosis (programmed cell death) in K562 leukemia cells. []
Q6: How is goniotriol synthesized?
A6: Goniotriol can be synthesized through various routes. One approach utilizes α-D-glucoheptonic-δ-lactone as the starting material, involving a multi-step process. [, ] Alternative strategies employ D-glycero-D-gulo-heptono-γ-lactone [] or a Julia–Colonna asymmetric epoxidation approach. []
Q7: What is the significance of the 8R configuration in goniotriol?
A7: Research suggests that the 8R configuration of goniotriol is crucial for its biological activity. Derivatives with modifications at this position often exhibit diminished antitumor activity compared to the parent compound. []
Q8: How do structural modifications impact the antitumor activity of goniotriol?
A8: Studies on goniotriol derivatives indicate that the unsaturated ester moiety of goniotriol plays a significant role in its antitumor activity. [] Modifications to this region, such as variations in the ester chain length and substituents, can significantly influence potency. []
Q9: Have any 3D-QSAR studies been conducted on goniotriol?
A9: Yes, 3D-QSAR (Quantitative Structure-Activity Relationship) studies using CoMFA (Comparative Molecular Field Analysis) have been employed to investigate the relationship between the structure of goniotriol derivatives and their activity against A2780 tumor cells. These models provide valuable insights into the steric and electrostatic features influencing goniotriol's antitumor activity. []
Disclaimer and Information on In-Vitro Research Products
Please be aware that all articles and product information presented on BenchChem are intended solely for informational purposes. The products available for purchase on BenchChem are specifically designed for in-vitro studies, which are conducted outside of living organisms. In-vitro studies, derived from the Latin term "in glass," involve experiments performed in controlled laboratory settings using cells or tissues. It is important to note that these products are not categorized as medicines or drugs, and they have not received approval from the FDA for the prevention, treatment, or cure of any medical condition, ailment, or disease. We must emphasize that any form of bodily introduction of these products into humans or animals is strictly prohibited by law. It is essential to adhere to these guidelines to ensure compliance with legal and ethical standards in research and experimentation.