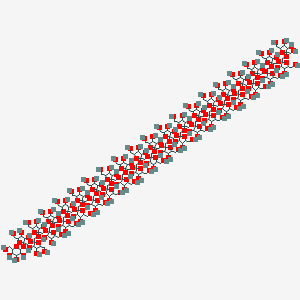
Inulin
Overview
Description
Inulin is a naturally occurring polysaccharide produced by many types of plantsThis compound is primarily composed of fructose units linked together by beta (2-1) glycosidic bonds, with a glucose molecule at the end of the chain . It is found in various plants, including chicory root, Jerusalem artichoke, and dandelion root . This compound serves as an energy reserve for plants and is used to regulate cold resistance . It is also recognized for its prebiotic properties, promoting the growth of beneficial gut bacteria .
Mechanism of Action
Target of Action
Inulin primarily targets the glomeruli , the main filtering structures of the kidney . It is used as a diagnostic agent to determine the rate of glomerular function . This compound is also known to interact with the gut microbiota, influencing its composition and activity .
Mode of Action
As a diagnostic agent, this compound is readily soluble and essentially indigestible . It readily passes through the blood and into the urine . It is neither secreted nor reabsorbed by the kidney, making it an excellent indicator for renal clearance rates . This compound is also a hypoglycemic agent .
Biochemical Pathways
This compound influences several biochemical pathways. As a diagnostic agent, it is used to measure the rate at which it is cleared from blood plasma, providing an indication of the filtering capacity of the glomeruli . As a nutraceutical agent, inulins may have antitumor, antimicrobial, hypolipidemic, and hypoglycemic actions . They may also help to improve mineral absorption and balance and may have antiosteoporotic activity .
Pharmacokinetics
This compound’s pharmacokinetics involve its absorption, distribution, and excretion . As a diagnostic agent, this compound is readily soluble and essentially indigestible . It readily passes through the blood and into the urine . It is neither secreted nor reabsorbed by the kidney, making it an excellent indicator for renal clearance rates .
Result of Action
The primary result of this compound’s action is the measurement of the rate of glomerular function . This is achieved by measuring the rate at which this compound, the test substance, is cleared from blood plasma . As a nutraceutical agent, inulins may have antitumor, antimicrobial, hypolipidemic, and hypoglycemic actions . They may also help to improve mineral absorption and balance and may have antiosteoporotic activity .
Action Environment
The action of this compound can be influenced by environmental factors. For example, the safety of this compound and sinistrin was questioned due to several reports of serious hypersensitivity reactions, including a fatal outcome . These reactions were associated with a specific batch of sinistrin . In general, this compound is considered safe and effective in both experimental animals and humans .
Biochemical Analysis
Biochemical Properties
Inulin interacts with various enzymes, proteins, and other biomolecules in the body. It is soluble in hot water and solutions of dilute acids and alkalis, and slightly soluble in cold water and organic solvents . The biochemical reactions involving this compound are complex and involve multiple steps, similar to a waterfall . The first step usually cannot be reversed, while other steps can be reversed depending on the cell’s needs .
Cellular Effects
This compound has significant effects on various types of cells and cellular processes. It influences cell function by changing their structures and stabilities in response to the surrounding environments . For instance, it can affect the concentration of associated proteins, RNA, and DNA, resulting in the formation of distinct subnuclear structures .
Molecular Mechanism
The molecular mechanism of this compound’s action involves its interactions with biomolecules and changes in gene expression. It exerts its effects at the molecular level through binding interactions with biomolecules, enzyme inhibition or activation . The exact mechanism of action is complex and involves multiple steps, each of which contributes to the overall effect of this compound on the body.
Temporal Effects in Laboratory Settings
In laboratory settings, the effects of this compound can change over time. This includes information on this compound’s stability, degradation, and any long-term effects on cellular function observed in in vitro or in vivo studies . The temporal effects of this compound are an important aspect of its biochemical profile and contribute to its overall impact on the body.
Dosage Effects in Animal Models
The effects of this compound vary with different dosages in animal models . These studies have observed threshold effects, as well as toxic or adverse effects at high doses. The exact dosage effects can vary depending on the specific animal model and the conditions of the study.
Metabolic Pathways
This compound is involved in various metabolic pathways in the body . It interacts with enzymes and cofactors, and can also affect metabolic flux or metabolite levels. The exact metabolic pathways that this compound is involved in can vary depending on the specific conditions of the body.
Transport and Distribution
This compound is transported and distributed within cells and tissues in the body . It interacts with transporters or binding proteins, and can also affect its localization or accumulation. The exact transport and distribution mechanisms of this compound can vary depending on the specific conditions of the body.
Subcellular Localization
The subcellular localization of this compound and its effects on its activity or function are important aspects of its biochemical profile . This could include any targeting signals or post-translational modifications that direct it to specific compartments or organelles. The exact subcellular localization of this compound can vary depending on the specific conditions of the body.
Preparation Methods
Synthetic Routes and Reaction Conditions: Inulin can be synthesized through enzymatic processes involving the polymerization of fructose units. The degree of polymerization can vary, resulting in different lengths of this compound chains .
Industrial Production Methods: Industrial production of this compound typically involves extraction from plant sources such as chicory root. The process includes the following steps:
Extraction: The plant material is washed, sliced, and subjected to hot water extraction to release this compound.
Purification: The extract is purified using techniques such as cellulose and dextran gel column chromatography to obtain high-purity this compound.
Drying and Milling: The purified this compound is dried and milled into a fine powder for various applications.
Chemical Reactions Analysis
Types of Reactions: Inulin undergoes several types of chemical reactions, including hydrolysis, oxidation, and polymerization .
Common Reagents and Conditions:
Hydrolysis: this compound can be hydrolyzed by acids or enzymes to produce fructose and glucose.
Oxidation: this compound can be oxidized using oxidizing agents to produce various derivatives.
Polymerization: this compound can be polymerized to form this compound-based hydrogels through methods such as methacrylate modification and free-radical polymerization.
Major Products:
Scientific Research Applications
Inulin has a wide range of scientific research applications:
Chemistry: this compound is used as a precursor for the synthesis of various chemicals and materials.
Medicine: this compound is used as a dietary fiber to improve digestive health, enhance mineral absorption, and support immune function.
Industry: this compound is used in the food industry as a fat substitute, thickener, and stabilizer.
Comparison with Similar Compounds
Oligofructose: A shorter-chain fructan with similar prebiotic properties.
Fructooligosaccharides: Short-chain fructans with similar health benefits but different degrees of polymerization.
Uniqueness of Inulin: this compound’s unique properties include its longer chain length, which provides a more sustained prebiotic effect compared to shorter-chain fructans . Additionally, this compound’s ability to form gels and its resistance to digestion make it a versatile ingredient in various applications .
Properties
IUPAC Name |
2-[2-[[2-[[2-[[2-[[2-[[2-[[2-[[2-[[2-[[2-[[2-[[2-[[2-[[2-[[2-[[2-[[2-[[2-[[2-[[2-[[2-[[2-[[2-[[2-[[2-[[2-[[2-[[2-[[2-[[2-[[2-[[2-[[2-[[2-[[2-[[2-[[3,4-dihydroxy-2,5-bis(hydroxymethyl)oxolan-2-yl]oxymethyl]-3,4-dihydroxy-5-(hydroxymethyl)oxolan-2-yl]oxymethyl]-3,4-dihydroxy-5-(hydroxymethyl)oxolan-2-yl]oxymethyl]-3,4-dihydroxy-5-(hydroxymethyl)oxolan-2-yl]oxymethyl]-3,4-dihydroxy-5-(hydroxymethyl)oxolan-2-yl]oxymethyl]-3,4-dihydroxy-5-(hydroxymethyl)oxolan-2-yl]oxymethyl]-3,4-dihydroxy-5-(hydroxymethyl)oxolan-2-yl]oxymethyl]-3,4-dihydroxy-5-(hydroxymethyl)oxolan-2-yl]oxymethyl]-3,4-dihydroxy-5-(hydroxymethyl)oxolan-2-yl]oxymethyl]-3,4-dihydroxy-5-(hydroxymethyl)oxolan-2-yl]oxymethyl]-3,4-dihydroxy-5-(hydroxymethyl)oxolan-2-yl]oxymethyl]-3,4-dihydroxy-5-(hydroxymethyl)oxolan-2-yl]oxymethyl]-3,4-dihydroxy-5-(hydroxymethyl)oxolan-2-yl]oxymethyl]-3,4-dihydroxy-5-(hydroxymethyl)oxolan-2-yl]oxymethyl]-3,4-dihydroxy-5-(hydroxymethyl)oxolan-2-yl]oxymethyl]-3,4-dihydroxy-5-(hydroxymethyl)oxolan-2-yl]oxymethyl]-3,4-dihydroxy-5-(hydroxymethyl)oxolan-2-yl]oxymethyl]-3,4-dihydroxy-5-(hydroxymethyl)oxolan-2-yl]oxymethyl]-3,4-dihydroxy-5-(hydroxymethyl)oxolan-2-yl]oxymethyl]-3,4-dihydroxy-5-(hydroxymethyl)oxolan-2-yl]oxymethyl]-3,4-dihydroxy-5-(hydroxymethyl)oxolan-2-yl]oxymethyl]-3,4-dihydroxy-5-(hydroxymethyl)oxolan-2-yl]oxymethyl]-3,4-dihydroxy-5-(hydroxymethyl)oxolan-2-yl]oxymethyl]-3,4-dihydroxy-5-(hydroxymethyl)oxolan-2-yl]oxymethyl]-3,4-dihydroxy-5-(hydroxymethyl)oxolan-2-yl]oxymethyl]-3,4-dihydroxy-5-(hydroxymethyl)oxolan-2-yl]oxymethyl]-3,4-dihydroxy-5-(hydroxymethyl)oxolan-2-yl]oxymethyl]-3,4-dihydroxy-5-(hydroxymethyl)oxolan-2-yl]oxymethyl]-3,4-dihydroxy-5-(hydroxymethyl)oxolan-2-yl]oxymethyl]-3,4-dihydroxy-5-(hydroxymethyl)oxolan-2-yl]oxymethyl]-3,4-dihydroxy-5-(hydroxymethyl)oxolan-2-yl]oxymethyl]-3,4-dihydroxy-5-(hydroxymethyl)oxolan-2-yl]oxymethyl]-3,4-dihydroxy-5-(hydroxymethyl)oxolan-2-yl]oxymethyl]-3,4-dihydroxy-5-(hydroxymethyl)oxolan-2-yl]oxymethyl]-3,4-dihydroxy-5-(hydroxymethyl)oxolan-2-yl]oxymethyl]-3,4-dihydroxy-5-(hydroxymethyl)oxolan-2-yl]oxymethyl]-3,4-dihydroxy-5-(hydroxymethyl)oxolan-2-yl]oxy-6-(hydroxymethyl)oxane-3,4,5-triol | |
---|---|---|
Source | PubChem | |
URL | https://pubchem.ncbi.nlm.nih.gov | |
Description | Data deposited in or computed by PubChem | |
InChI |
InChI=1S/C228H382O191/c229-1-76-114(268)152(306)153(307)191(381-76)419-228(190(344)151(305)113(38-266)418-228)75-380-227(189(343)150(304)112(37-265)417-227)74-379-226(188(342)149(303)111(36-264)416-226)73-378-225(187(341)148(302)110(35-263)415-225)72-377-224(186(340)147(301)109(34-262)414-224)71-376-223(185(339)146(300)108(33-261)413-223)70-375-222(184(338)145(299)107(32-260)412-222)69-374-221(183(337)144(298)106(31-259)411-221)68-373-220(182(336)143(297)105(30-258)410-220)67-372-219(181(335)142(296)104(29-257)409-219)66-371-218(180(334)141(295)103(28-256)408-218)65-370-217(179(333)140(294)102(27-255)407-217)64-369-216(178(332)139(293)101(26-254)406-216)63-368-215(177(331)138(292)100(25-253)405-215)62-367-214(176(330)137(291)99(24-252)404-214)61-366-213(175(329)136(290)98(23-251)403-213)60-365-212(174(328)135(289)97(22-250)402-212)59-364-211(173(327)134(288)96(21-249)401-211)58-363-210(172(326)133(287)95(20-248)400-210)57-362-209(171(325)132(286)94(19-247)399-209)56-361-208(170(324)131(285)93(18-246)398-208)55-360-207(169(323)130(284)92(17-245)397-207)54-359-206(168(322)129(283)91(16-244)396-206)53-358-205(167(321)128(282)90(15-243)395-205)52-357-204(166(320)127(281)89(14-242)394-204)51-356-203(165(319)126(280)88(13-241)393-203)50-355-202(164(318)125(279)87(12-240)392-202)49-354-201(163(317)124(278)86(11-239)391-201)48-353-200(162(316)123(277)85(10-238)390-200)47-352-199(161(315)122(276)84(9-237)389-199)46-351-198(160(314)121(275)83(8-236)388-198)45-350-197(159(313)120(274)82(7-235)387-197)44-349-196(158(312)119(273)81(6-234)386-196)43-348-195(157(311)118(272)80(5-233)385-195)42-347-194(156(310)117(271)79(4-232)384-194)41-346-193(155(309)116(270)78(3-231)383-193)40-345-192(39-267)154(308)115(269)77(2-230)382-192/h76-191,229-344H,1-75H2 | |
Source | PubChem | |
URL | https://pubchem.ncbi.nlm.nih.gov | |
Description | Data deposited in or computed by PubChem | |
InChI Key |
JYJIGFIDKWBXDU-UHFFFAOYSA-N | |
Source | PubChem | |
URL | https://pubchem.ncbi.nlm.nih.gov | |
Description | Data deposited in or computed by PubChem | |
Canonical SMILES |
C(C1C(C(C(C(O1)OC2(C(C(C(O2)CO)O)O)COC3(C(C(C(O3)CO)O)O)COC4(C(C(C(O4)CO)O)O)COC5(C(C(C(O5)CO)O)O)COC6(C(C(C(O6)CO)O)O)COC7(C(C(C(O7)CO)O)O)COC8(C(C(C(O8)CO)O)O)COC9(C(C(C(O9)CO)O)O)COC1(C(C(C(O1)CO)O)O)COC1(C(C(C(O1)CO)O)O)COC1(C(C(C(O1)CO)O)O)COC1(C(C(C(O1)CO)O)O)COC1(C(C(C(O1)CO)O)O)COC1(C(C(C(O1)CO)O)O)COC1(C(C(C(O1)CO)O)O)COC1(C(C(C(O1)CO)O)O)COC1(C(C(C(O1)CO)O)O)COC1(C(C(C(O1)CO)O)O)COC1(C(C(C(O1)CO)O)O)COC1(C(C(C(O1)CO)O)O)COC1(C(C(C(O1)CO)O)O)COC1(C(C(C(O1)CO)O)O)COC1(C(C(C(O1)CO)O)O)COC1(C(C(C(O1)CO)O)O)COC1(C(C(C(O1)CO)O)O)COC1(C(C(C(O1)CO)O)O)COC1(C(C(C(O1)CO)O)O)COC1(C(C(C(O1)CO)O)O)COC1(C(C(C(O1)CO)O)O)COC1(C(C(C(O1)CO)O)O)COC1(C(C(C(O1)CO)O)O)COC1(C(C(C(O1)CO)O)O)COC1(C(C(C(O1)CO)O)O)COC1(C(C(C(O1)CO)O)O)COC1(C(C(C(O1)CO)O)O)COC1(C(C(C(O1)CO)O)O)COC1(C(C(C(O1)CO)O)O)CO)O)O)O)O | |
Source | PubChem | |
URL | https://pubchem.ncbi.nlm.nih.gov | |
Description | Data deposited in or computed by PubChem | |
Molecular Formula |
C228H382O191 | |
Source | PubChem | |
URL | https://pubchem.ncbi.nlm.nih.gov | |
Description | Data deposited in or computed by PubChem | |
Molecular Weight |
6179 g/mol | |
Source | PubChem | |
URL | https://pubchem.ncbi.nlm.nih.gov | |
Description | Data deposited in or computed by PubChem | |
Physical Description |
Solid | |
Record name | Inulin | |
Source | Human Metabolome Database (HMDB) | |
URL | http://www.hmdb.ca/metabolites/HMDB0014776 | |
Description | The Human Metabolome Database (HMDB) is a freely available electronic database containing detailed information about small molecule metabolites found in the human body. | |
Explanation | HMDB is offered to the public as a freely available resource. Use and re-distribution of the data, in whole or in part, for commercial purposes requires explicit permission of the authors and explicit acknowledgment of the source material (HMDB) and the original publication (see the HMDB citing page). We ask that users who download significant portions of the database cite the HMDB paper in any resulting publications. | |
CAS No. |
9005-80-5 | |
Record name | Inulin | |
Source | Human Metabolome Database (HMDB) | |
URL | http://www.hmdb.ca/metabolites/HMDB0014776 | |
Description | The Human Metabolome Database (HMDB) is a freely available electronic database containing detailed information about small molecule metabolites found in the human body. | |
Explanation | HMDB is offered to the public as a freely available resource. Use and re-distribution of the data, in whole or in part, for commercial purposes requires explicit permission of the authors and explicit acknowledgment of the source material (HMDB) and the original publication (see the HMDB citing page). We ask that users who download significant portions of the database cite the HMDB paper in any resulting publications. | |
Melting Point |
178 °C | |
Record name | Inulin | |
Source | Human Metabolome Database (HMDB) | |
URL | http://www.hmdb.ca/metabolites/HMDB0014776 | |
Description | The Human Metabolome Database (HMDB) is a freely available electronic database containing detailed information about small molecule metabolites found in the human body. | |
Explanation | HMDB is offered to the public as a freely available resource. Use and re-distribution of the data, in whole or in part, for commercial purposes requires explicit permission of the authors and explicit acknowledgment of the source material (HMDB) and the original publication (see the HMDB citing page). We ask that users who download significant portions of the database cite the HMDB paper in any resulting publications. | |
Disclaimer and Information on In-Vitro Research Products
Please be aware that all articles and product information presented on BenchChem are intended solely for informational purposes. The products available for purchase on BenchChem are specifically designed for in-vitro studies, which are conducted outside of living organisms. In-vitro studies, derived from the Latin term "in glass," involve experiments performed in controlled laboratory settings using cells or tissues. It is important to note that these products are not categorized as medicines or drugs, and they have not received approval from the FDA for the prevention, treatment, or cure of any medical condition, ailment, or disease. We must emphasize that any form of bodily introduction of these products into humans or animals is strictly prohibited by law. It is essential to adhere to these guidelines to ensure compliance with legal and ethical standards in research and experimentation.