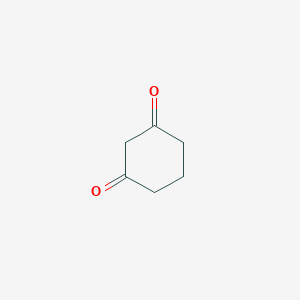
1,3-Cyclohexanedione
Overview
Description
1,3-Cyclohexanedione is an organic compound with the molecular formula C6H8O2. It is one of three isomeric cyclohexanediones and is known for its colorless or white solid appearance. This compound occurs naturally and is a substrate for cyclohexanedione hydrolase. It predominantly exists in its enol tautomer form .
Mechanism of Action
Target of Action
1,3-Cyclohexanedione primarily targets the enzyme 4-Hydroxyphenylpyruvate dioxygenase (HPPD) . HPPD is a key enzyme in the development of herbicides and plays a crucial role in the degradation of the amino acid tyrosine .
Mode of Action
This compound interacts with its target, HPPD, by inhibiting its activity . This inhibition disrupts the normal synthesis of Homogentisic Acid (HGA), a precursor to the synthetic plastoquinone and tocopherol, which are vital for photosynthesis . The inhibition of HPPD leads to the failure of the normal synthesis of HGA, which results in the obstruction of the synthesis of plastoquinone, affects the photosynthesis of plants, and causes the death of plants with bleaching symptoms .
Biochemical Pathways
The primary biochemical pathway affected by this compound is the tyrosine degradation pathway . This pathway involves the conversion of 4-hydroxyphenylpyruvate (HPPA) into homogentisic acid (HGA) in plants . When HPPD is inhibited by this compound, the normal synthesis of HGA fails, obstructing the synthesis of plastoquinone and affecting photosynthesis .
Pharmacokinetics
It’s known that the compound can be analyzed using reverse phase (rp) hplc method with simple conditions . More research is needed to fully understand the ADME properties of this compound and their impact on its bioavailability.
Result of Action
The primary result of this compound’s action is the inhibition of photosynthesis in plants, leading to plant death with bleaching symptoms . This makes this compound an effective herbicide, particularly against certain types of weeds .
Action Environment
The effectiveness of this compound can be influenced by various environmental factors. For instance, the compound’s herbicidal activity was evaluated against Brassia campestris (BC) and Echinochloa crus-galli (EC) at a dosage of 225 g ai/ha . .
Biochemical Analysis
Biochemical Properties
1,3-Cyclohexanedione is the substrate for cyclohexanedione hydrolase . It reacts under acid catalysis with alcohols to 3-alkoxyenones . Its pKa is 5.26 .
Molecular Mechanism
This compound exists in solution predominantly as the enol tautomer . It reacts under acid catalysis with alcohols to 3-alkoxyenones . Treatment of the sodium salt of the enolate with methyl iodide gives 2-methyl-1,3-cyclohexanedione, which also exists predominantly as the enol .
Metabolic Pathways
This compound is involved in the metabolic pathway where it is the substrate for cyclohexanedione hydrolase
Preparation Methods
1,3-Cyclohexanedione can be synthesized through several methods:
Semi-hydrogenation of Resorcinol: This method involves the hydrogenation of resorcinol (C6H4(OH)2) in the presence of a catalyst to produce this compound.
Tetraacetylethylene Treatment: Tetraacetylethylene is treated with 1,5-diazabicyclo[4.3.0]non-5-ene or sodium acetate to form cyclopentenone, which is then converted to this compound.
Industrial Production: In industrial settings, resorcinol is neutralized with sodium hydroxide and then hydrogenated at high pressure and temperature.
Chemical Reactions Analysis
1,3-Cyclohexanedione undergoes various chemical reactions:
Enolization: It predominantly exists as the enol tautomer in solution.
Acid-Catalyzed Reactions: Under acid catalysis, it reacts with alcohols to form 3-alkoxyenones.
Alkylation: The sodium salt of the enolate reacts with methyl iodide to produce 2-methyl-1,3-cyclohexanedione.
Nitrosation: It can be nitrosated to form 1,2,3-cyclohexanetrione 2-oxime.
Reduction and Substitution: It reacts with various reagents to form different derivatives, such as 3-undecanoyloxy-2-cyclohexen-1-one and 2-bromo-4-undecyl-1,3-cyclohexanedione.
Scientific Research Applications
1,3-Cyclohexanedione has numerous applications in scientific research:
Comparison with Similar Compounds
1,3-Cyclohexanedione can be compared with other similar compounds:
Dimedone (5,5-dimethyl-1,3-cyclohexanedione): A well-established reagent used in various chemical reactions.
1,2,3-Cyclohexanetrione: Similar in chemical properties but less stable than this compound.
1,4-Cyclohexanedione: Another isomer with different reactivity and applications.
This compound stands out due to its versatility in forming various derivatives and its significant role in the synthesis of herbicides and other industrial chemicals.
Properties
IUPAC Name |
cyclohexane-1,3-dione | |
---|---|---|
Source | PubChem | |
URL | https://pubchem.ncbi.nlm.nih.gov | |
Description | Data deposited in or computed by PubChem | |
InChI |
InChI=1S/C6H8O2/c7-5-2-1-3-6(8)4-5/h1-4H2 | |
Source | PubChem | |
URL | https://pubchem.ncbi.nlm.nih.gov | |
Description | Data deposited in or computed by PubChem | |
InChI Key |
HJSLFCCWAKVHIW-UHFFFAOYSA-N | |
Source | PubChem | |
URL | https://pubchem.ncbi.nlm.nih.gov | |
Description | Data deposited in or computed by PubChem | |
Canonical SMILES |
C1CC(=O)CC(=O)C1 | |
Source | PubChem | |
URL | https://pubchem.ncbi.nlm.nih.gov | |
Description | Data deposited in or computed by PubChem | |
Molecular Formula |
C6H8O2 | |
Source | PubChem | |
URL | https://pubchem.ncbi.nlm.nih.gov | |
Description | Data deposited in or computed by PubChem | |
DSSTOX Substance ID |
DTXSID1044433 | |
Record name | 1,3-Cyclohexanedione | |
Source | EPA DSSTox | |
URL | https://comptox.epa.gov/dashboard/DTXSID1044433 | |
Description | DSSTox provides a high quality public chemistry resource for supporting improved predictive toxicology. | |
Molecular Weight |
112.13 g/mol | |
Source | PubChem | |
URL | https://pubchem.ncbi.nlm.nih.gov | |
Description | Data deposited in or computed by PubChem | |
Physical Description |
Dry Powder, Solid; [Merck Index] Light brown powder; [Alfa Aesar MSDS] | |
Record name | 1,3-Cyclohexanedione | |
Source | EPA Chemicals under the TSCA | |
URL | https://www.epa.gov/chemicals-under-tsca | |
Description | EPA Chemicals under the Toxic Substances Control Act (TSCA) collection contains information on chemicals and their regulations under TSCA, including non-confidential content from the TSCA Chemical Substance Inventory and Chemical Data Reporting. | |
Record name | 1,3-Cyclohexanedione | |
Source | Haz-Map, Information on Hazardous Chemicals and Occupational Diseases | |
URL | https://haz-map.com/Agents/9767 | |
Description | Haz-Map® is an occupational health database designed for health and safety professionals and for consumers seeking information about the adverse effects of workplace exposures to chemical and biological agents. | |
Explanation | Copyright (c) 2022 Haz-Map(R). All rights reserved. Unless otherwise indicated, all materials from Haz-Map are copyrighted by Haz-Map(R). No part of these materials, either text or image may be used for any purpose other than for personal use. Therefore, reproduction, modification, storage in a retrieval system or retransmission, in any form or by any means, electronic, mechanical or otherwise, for reasons other than personal use, is strictly prohibited without prior written permission. | |
CAS No. |
504-02-9 | |
Record name | 1,3-Cyclohexanedione | |
Source | CAS Common Chemistry | |
URL | https://commonchemistry.cas.org/detail?cas_rn=504-02-9 | |
Description | CAS Common Chemistry is an open community resource for accessing chemical information. Nearly 500,000 chemical substances from CAS REGISTRY cover areas of community interest, including common and frequently regulated chemicals, and those relevant to high school and undergraduate chemistry classes. This chemical information, curated by our expert scientists, is provided in alignment with our mission as a division of the American Chemical Society. | |
Explanation | The data from CAS Common Chemistry is provided under a CC-BY-NC 4.0 license, unless otherwise stated. | |
Record name | Dihydroresorcinol | |
Source | ChemIDplus | |
URL | https://pubchem.ncbi.nlm.nih.gov/substance/?source=chemidplus&sourceid=0000504029 | |
Description | ChemIDplus is a free, web search system that provides access to the structure and nomenclature authority files used for the identification of chemical substances cited in National Library of Medicine (NLM) databases, including the TOXNET system. | |
Record name | 1,3-Cyclohexanedione | |
Source | DTP/NCI | |
URL | https://dtp.cancer.gov/dtpstandard/servlet/dwindex?searchtype=NSC&outputformat=html&searchlist=57477 | |
Description | The NCI Development Therapeutics Program (DTP) provides services and resources to the academic and private-sector research communities worldwide to facilitate the discovery and development of new cancer therapeutic agents. | |
Explanation | Unless otherwise indicated, all text within NCI products is free of copyright and may be reused without our permission. Credit the National Cancer Institute as the source. | |
Record name | 1,3-Cyclohexanedione | |
Source | EPA Chemicals under the TSCA | |
URL | https://www.epa.gov/chemicals-under-tsca | |
Description | EPA Chemicals under the Toxic Substances Control Act (TSCA) collection contains information on chemicals and their regulations under TSCA, including non-confidential content from the TSCA Chemical Substance Inventory and Chemical Data Reporting. | |
Record name | 1,3-Cyclohexanedione | |
Source | EPA DSSTox | |
URL | https://comptox.epa.gov/dashboard/DTXSID1044433 | |
Description | DSSTox provides a high quality public chemistry resource for supporting improved predictive toxicology. | |
Record name | Cyclohexane-1,3-dione | |
Source | European Chemicals Agency (ECHA) | |
URL | https://echa.europa.eu/substance-information/-/substanceinfo/100.007.255 | |
Description | The European Chemicals Agency (ECHA) is an agency of the European Union which is the driving force among regulatory authorities in implementing the EU's groundbreaking chemicals legislation for the benefit of human health and the environment as well as for innovation and competitiveness. | |
Explanation | Use of the information, documents and data from the ECHA website is subject to the terms and conditions of this Legal Notice, and subject to other binding limitations provided for under applicable law, the information, documents and data made available on the ECHA website may be reproduced, distributed and/or used, totally or in part, for non-commercial purposes provided that ECHA is acknowledged as the source: "Source: European Chemicals Agency, http://echa.europa.eu/". Such acknowledgement must be included in each copy of the material. ECHA permits and encourages organisations and individuals to create links to the ECHA website under the following cumulative conditions: Links can only be made to webpages that provide a link to the Legal Notice page. | |
Record name | DIHYDRORESORCINOL | |
Source | FDA Global Substance Registration System (GSRS) | |
URL | https://gsrs.ncats.nih.gov/ginas/app/beta/substances/6UK3D2BXJT | |
Description | The FDA Global Substance Registration System (GSRS) enables the efficient and accurate exchange of information on what substances are in regulated products. Instead of relying on names, which vary across regulatory domains, countries, and regions, the GSRS knowledge base makes it possible for substances to be defined by standardized, scientific descriptions. | |
Explanation | Unless otherwise noted, the contents of the FDA website (www.fda.gov), both text and graphics, are not copyrighted. They are in the public domain and may be republished, reprinted and otherwise used freely by anyone without the need to obtain permission from FDA. Credit to the U.S. Food and Drug Administration as the source is appreciated but not required. | |
Synthesis routes and methods
Procedure details
Retrosynthesis Analysis
AI-Powered Synthesis Planning: Our tool employs the Template_relevance Pistachio, Template_relevance Bkms_metabolic, Template_relevance Pistachio_ringbreaker, Template_relevance Reaxys, Template_relevance Reaxys_biocatalysis model, leveraging a vast database of chemical reactions to predict feasible synthetic routes.
One-Step Synthesis Focus: Specifically designed for one-step synthesis, it provides concise and direct routes for your target compounds, streamlining the synthesis process.
Accurate Predictions: Utilizing the extensive PISTACHIO, BKMS_METABOLIC, PISTACHIO_RINGBREAKER, REAXYS, REAXYS_BIOCATALYSIS database, our tool offers high-accuracy predictions, reflecting the latest in chemical research and data.
Strategy Settings
Precursor scoring | Relevance Heuristic |
---|---|
Min. plausibility | 0.01 |
Model | Template_relevance |
Template Set | Pistachio/Bkms_metabolic/Pistachio_ringbreaker/Reaxys/Reaxys_biocatalysis |
Top-N result to add to graph | 6 |
Feasible Synthetic Routes
Q1: What is 1,3-Cyclohexanedione and what are some of its key applications?
A1: this compound is a cyclic diketone that exists in equilibrium with its enol tautomers. It serves as a versatile building block in organic synthesis and is a key structural motif found in various natural products and pharmaceuticals. It has found applications in the development of insecticides, herbicides, fungicides, and plant growth regulators. []
Q2: What is the molecular formula and weight of this compound?
A2: The molecular formula of this compound is C6H8O2, and its molecular weight is 112.13 g/mol.
Q3: How can you differentiate between the keto and enol forms of this compound using spectroscopic techniques?
A3: Inner-shell spectroscopy, particularly C 1s and O 1s excitation spectra, provides a clear distinction. These spectra reveal specific features characteristic of diketo and monoenol structures. Studies using electron energy loss spectra have confirmed the predominance of the diketonic structure for this compound in the gas phase. []
Q4: How does the solvent affect the keto-enol tautomerism of this compound?
A4: The keto-enol ratio of this compound is highly dependent on the solvent. This dependence arises from variations in solute–solvent and solute–solute hydrogen bonding interactions. Low-temperature high-performance liquid chromatography (HPLC) studies have demonstrated this solvent-dependent tautomeric behavior. []
Q5: What are the main fragmentation pathways observed in the mass spectra of methyl enol ethers of 1,3-Cyclohexanediones?
A5: The fragmentation patterns of methyl enol ethers of 1,3-Cyclohexanediones are consistent with those expected for 1,3-cyclohexanediones in their enol form. These fragmentation pathways have been studied extensively using deuterium labeling and provide insights into the influence of alkyl substituents on their mass spectral behavior. []
Q6: How does the presence of alkyl substituents influence the mass spectral behavior of 1,3-Cyclopentanediones compared to their this compound counterparts?
A6: Unlike 1,3-Cyclohexanediones, the mass spectra of 4- or 5-substituted 1,3-Cyclopentanedione enol ethers are identical, suggesting isomerization. This phenomenon has been investigated through metastable transitions, low-energy spectra, and deuterium labeling, leading to a proposed mechanism for this isomerization process. []
Q7: What are some examples of reactions involving this compound that highlight its synthetic utility?
A7: this compound exhibits diverse reactivity:
- Aromatization: Reacting this compound with bis(trichloromethyl) carbonate (BTC) and N,N-dimethylformamide (DMF) can lead to the formation of polysubstituted chlorobenzaldehydes, valuable intermediates in various synthetic applications. []
- Condensation Reactions: It readily undergoes condensation with aromatic aldehydes, catalyzed by acids or bases, to form xanthenedione derivatives. These reactions have been successfully carried out using various catalysts, including acidic ionic liquids like 1-methyllimidazolium hydrogen sulphate ([Hmim]HS04), offering advantages such as environmental friendliness, ease of work-up, short reaction times, high yields, and reusability of the ionic liquid catalyst. [, ]
- Cycloadditions: Under solvent-free ball-milling conditions and mediated by manganese(III) acetate dihydrate, this compound participates in cycloadditions with 1-(pyridin-2-yl)-enones, generating trans-2-acyl-3-aryl/alkyl-2,3,6,7-tetrahydro-4(5H)-benzofuranone derivatives. These reactions exhibit high diastereoselectivity and unexpected regioselectivity, with manganese(III) acetate playing a dual role as both an oxidant and a Lewis acid. []
- Radical Reactions: In the presence of manganese(III) acetate, this compound undergoes radical reactions with in situ generated imines under solid-state conditions using mechanical milling. These reactions proceed efficiently at room temperature, leading to good to excellent yields of the desired products. []
Q8: Has this compound been investigated for its potential biological activity?
A8: Yes, this compound and its derivatives have shown interesting biological activities:
- Radioprotective Effects: 2-Methyl-1,3-cyclohexanedione exhibits protective effects against radiation damage in Escherichia coli. It safeguards the bacteria from the lethal impact of ultraviolet light and ⁶⁰Co-induced lethality. While the precise mechanisms of action remain unknown, this finding suggests potential applications in radioprotection. []
- Enzyme Inhibition: Derivatives like 2-(2-nitro-4-fluoromethylbenzoyl)-1,3-cyclohexanedione (NTBC) and mesotrione act as inhibitors of the enzyme 4-hydroxyphenylpyruvate dioxygenase (HPPD). NTBC has shown efficacy in treating hereditary tyrosinaemia type 1 (HT-1). []
Q9: How do the pharmacological properties of NTBC and mesotrione, both HPPD inhibitors, differ?
A9: Despite both being HPPD inhibitors, NTBC and mesotrione exhibit distinct pharmacological profiles. NTBC demonstrates a persistent and significant inhibitory effect on HPPD, resulting in a prolonged elevation of tyrosine levels in plasma. In contrast, mesotrione's effect on HPPD is transient and minimal, leading to a much smaller and shorter-lived increase in tyrosine concentrations. These differences in pharmacological behavior underscore their suitability for different applications. NTBC's long-lasting effect makes it suitable for treating HT-1, while mesotrione's minimal and transient effect minimizes the likelihood of adverse effects during occupational use as an herbicide. []
Q10: What analytical techniques are commonly employed for the characterization and quantification of this compound and its derivatives?
A10: Several analytical methods are routinely used:
- Chromatography: High-performance liquid chromatography (HPLC) enables the separation and quantification of this compound and its derivatives. When coupled with fluorescence detection, it offers sensitive detection of trace amounts, for example, in the analysis of formaldehyde in food samples after derivatization with this compound. []
Q11: Are there any documented phase transitions in this compound crystals?
A11: Yes, 2-methyl-1,3-cyclohexanedione crystals exhibit a second-order phase transition at 243 K. This transition has been studied using techniques such as Brillouin scattering, NMR, calorimetry, and dielectric permittivity measurements. The transition entropy has been determined to be 5.7 J mol⁻¹ K⁻¹, and NMR studies suggest two possible models for molecular reorientation during this phase change. Dielectric studies indicate an activation process around the transition temperature. Furthermore, Brillouin scattering measurements of elastic constants reveal that the phase transition in 2-methyl-1,3-cyclohexanedione crystals exhibits slow dynamics similar to an order-disorder transformation, without any softening of modes. This behavior contrasts with the phase transitions observed in the analogous compound, this compound. [, ]
Disclaimer and Information on In-Vitro Research Products
Please be aware that all articles and product information presented on BenchChem are intended solely for informational purposes. The products available for purchase on BenchChem are specifically designed for in-vitro studies, which are conducted outside of living organisms. In-vitro studies, derived from the Latin term "in glass," involve experiments performed in controlled laboratory settings using cells or tissues. It is important to note that these products are not categorized as medicines or drugs, and they have not received approval from the FDA for the prevention, treatment, or cure of any medical condition, ailment, or disease. We must emphasize that any form of bodily introduction of these products into humans or animals is strictly prohibited by law. It is essential to adhere to these guidelines to ensure compliance with legal and ethical standards in research and experimentation.