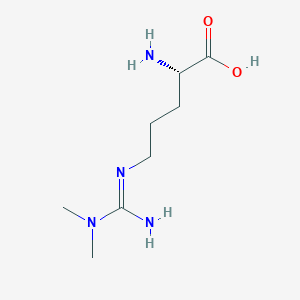
N,N-dimethylarginine
Overview
Description
N,N-Dimethylarginine is a naturally occurring chemical found in blood plasma. It is a metabolic by-product of continual protein modification processes in the cytoplasm of all human cells. This compound is closely related to L-arginine, a conditionally-essential amino acid. This compound interferes with L-arginine in the production of nitric oxide, a key chemical for endothelial and cardiovascular health .
Mechanism of Action
Target of Action
Asymmetric dimethylarginine (ADMA) is an endogenous inhibitor of nitric oxide synthase (NOS), an enzyme that plays a crucial role in promoting cardiovascular health . ADMA competes with L-arginine for binding to NOS, thereby inhibiting the production of nitric oxide (NO), a key chemical involved in normal endothelial function .
Mode of Action
ADMA interferes with L-arginine in the production of nitric oxide (NO). It is formed by methylation of arginine residues in proteins and released after proteolysis . In this reaction, S-adenosylmethionine acts as the methyl donor and S-adenosylhomocysteine is the demethylated product . This interaction results in decreased vasodilation, increased smooth muscle cell proliferation, platelet dysfunction, and increased monocyte adhesion .
Biochemical Pathways
ADMA is created in protein methylation, a common mechanism of post-translational protein modification. This reaction is catalyzed by an enzyme set called protein arginine N-methyltransferases 1 and 2 . The methyl groups transferred to create ADMA are derived from the methyl group donor S-adenosylmethionine, an intermediate in the metabolism of homocysteine . After synthesis, ADMA migrates into the extracellular space and thence into blood plasma .
Pharmacokinetics
ADMA is a naturally occurring chemical found in blood plasma. It is a metabolic by-product of continual protein modification processes in the cytoplasm of all human cells .
Result of Action
The inhibition of NO production by ADMA leads to impaired endothelial function, resulting in decreased vasodilation, increased smooth muscle cell proliferation, platelet dysfunction, and increased monocyte adhesion . Additionally, ADMA may contribute to oxidative stress by causing eNOS uncoupling via depletion of tetrahydrobiopterin (BH4), resulting in a shift from NO production to superoxide production .
Action Environment
The action of ADMA is influenced by various environmental factors. For instance, ADMA concentrations are substantially elevated by native or oxidized LDL cholesterol . Furthermore, the impact of ADMA on vascular resistance and the function of the heart can be influenced by various diagnosed diseases .
Biochemical Analysis
Biochemical Properties
ADMA is an endogenous inhibitor of nitric oxide synthase . It is related to endothelial dysfunction, which plays an important role in vascular damage elicited by various cardiometabolic risk factors . It is formed by methylation of arginine residues in proteins and released after proteolysis .
Cellular Effects
ADMA interferes with the production of nitric oxide, a key chemical involved in normal endothelial function and, by extension, cardiovascular health . Elevated ADMA levels have been associated with cardiovascular disease in renal failure patients . Both homocysteine and ADMA are thought to mediate their adverse vascular effects by impairing endothelial, nitric oxide-dependent function resulting in decreased vasodilatation, increased smooth muscle cell proliferation, platelet dysfunction, and increased monocyte adhesion .
Molecular Mechanism
ADMA, an endogenous inhibitor of nitric oxide synthase, is formed by methylation of arginine residues in proteins and released after proteolysis . In this reaction, S-adenosylmethionine is the methyl donor and S-adenosylhomocysteine the demethylated product .
Temporal Effects in Laboratory Settings
It is known that ADMA is a stable compound that can be measured using high-performance liquid chromatography .
Dosage Effects in Animal Models
It is known that overexpression of dimethylarginine dimethylaminohydrolase 1 (DDAH1), an enzyme degrading ADMA, exerts protective effects in animal models .
Metabolic Pathways
ADMA is created in protein methylation, a common mechanism of post-translational protein modification . This reaction is catalyzed by an enzyme set called protein arginine N-methyltransferases 1 and 2 . The methyl groups transferred to create ADMA are derived from the methyl group donor S-adenosylmethionine, an intermediate in the metabolism of homocysteine .
Transport and Distribution
The mitochondrial solute carrier SLC25A2 transports ADMA across the liposomal membrane in both directions by both unidirectional transport and exchange against arginine or lysine . The SLC25A2-mediated ADMA transport followed first-order kinetics .
Subcellular Localization
It is known that ADMA is a naturally occurring chemical found in blood plasma , suggesting that it is present in the cytoplasm of cells
Preparation Methods
Synthetic Routes and Reaction Conditions: N,N-Dimethylarginine is synthesized through the methylation of arginine residues in proteins. This process involves the enzyme protein arginine methyltransferase, which uses S-adenosylmethionine as the methyl donor. The methylation occurs post-translationally, and the methylated arginine residues are released after proteolysis .
Industrial Production Methods: Industrial production of this compound typically involves the use of high-performance liquid chromatography (HPLC) and mass spectrometry (MS) for its quantification and purification. These methods ensure the precise measurement and separation of this compound from other similar compounds .
Chemical Reactions Analysis
Types of Reactions: N,N-Dimethylarginine undergoes several types of chemical reactions, including:
Oxidation: This reaction involves the conversion of this compound to other nitrogenous compounds.
Reduction: This reaction can lead to the formation of simpler nitrogenous compounds.
Substitution: This reaction involves the replacement of one functional group with another.
Common Reagents and Conditions:
Oxidation: Common reagents include oxidizing agents such as hydrogen peroxide.
Reduction: Reducing agents like sodium borohydride are often used.
Substitution: Various nucleophiles can be used depending on the desired product.
Major Products Formed: The major products formed from these reactions include citrulline and dimethylamine, which are produced through the enzymatic hydrolysis of this compound .
Scientific Research Applications
Cardiovascular Research
Role as a Biomarker:
ADMA is increasingly recognized as a biomarker for cardiovascular diseases. Elevated levels of ADMA have been associated with several conditions, including hypertension, atherosclerosis, and chronic heart failure. Studies indicate that plasma ADMA concentrations are significantly higher in patients with end-stage renal disease compared to healthy individuals, suggesting its potential utility in risk stratification and monitoring disease progression .
Mechanisms of Action:
ADMA inhibits endothelial nitric oxide production, which can lead to vascular dysfunction. In vitro studies have demonstrated that ADMA can increase superoxide production in endothelial cells, activating redox-sensitive transcription factors like NF-κB and promoting inflammation . This mechanism underpins its role in cardiovascular pathologies.
Renal Function and Disease
Impact on Kidney Health:
Research has shown that elevated ADMA levels are linked to renal impairment. In patients with chronic kidney disease, ADMA concentrations correlate with the severity of the disease. The inhibition of NOS by ADMA contributes to endothelial dysfunction, which is a common complication in renal patients .
Therapeutic Potential:
Interventions aimed at reducing ADMA levels may improve renal outcomes. For instance, studies suggest that periodontal treatments can lower ADMA levels, potentially enhancing endothelial function in patients with comorbidities such as chronic kidney disease .
Pediatric Applications
Clinical Insights:
The role of ADMA in pediatric populations is an emerging area of research. Elevated ADMA levels have been implicated in various childhood diseases, particularly those affecting cardiovascular health. Understanding age-specific reference ranges for ADMA is crucial for its application as a biomarker in pediatric medicine .
Future Directions:
There is potential for developing therapeutic strategies targeting ADMA reduction in children with cardiovascular risk factors. Ongoing research aims to clarify the implications of elevated ADMA in growth and development among pediatric patients .
Periodontal Disease and Systemic Health
Link Between Periodontitis and Cardiovascular Disease:
Recent studies have identified a significant association between periodontal disease and elevated ADMA levels, suggesting that inflammation from periodontal conditions may contribute to systemic vascular dysfunction .
Clinical Interventions:
Periodontal treatments have been shown to effectively reduce ADMA levels, indicating that managing oral health may have beneficial effects on cardiovascular health by improving endothelial function .
COVID-19 Research
Potential Role in Disease Severity:
Research into the role of ADMA during COVID-19 has suggested that elevated levels may be linked to worse respiratory outcomes and pulmonary vasoregulation issues. Understanding how ADMA influences nitric oxide pathways during viral infections could provide insights into therapeutic targets for managing severe cases .
Data Summary Table
Comparison with Similar Compounds
Symmetric Dimethylarginine: This compound is also a methylated derivative of arginine but differs in its methylation pattern.
NG-Monomethylarginine: Another methylated arginine derivative with a single methyl group.
Uniqueness: N,N-Dimethylarginine is unique due to its asymmetric methylation, which specifically inhibits nitric oxide synthase, whereas symmetric dimethylarginine does not have this inhibitory effect .
Biological Activity
N,N-Dimethylarginine (often referred to as asymmetric dimethylarginine or ADMA) is a naturally occurring amino acid derivative that plays a crucial role in various biological processes, particularly in the regulation of nitric oxide (NO) synthesis. Its biological activity is primarily characterized by its function as an endogenous inhibitor of nitric oxide synthase (NOS), which has significant implications for cardiovascular health and other physiological functions.
Overview of this compound
This compound is produced through the methylation of L-arginine, a process facilitated by protein arginine methyltransferases (PRMTs). This compound exists in two forms: asymmetric dimethylarginine (ADMA) and symmetric dimethylarginine (SDMA). Among these, ADMA is the more biologically active form, known for its inhibitory effects on NOS, which reduces the bioavailability of NO—a key vasodilator involved in maintaining vascular tone and homeostasis.
ADMA competes with L-arginine for binding to NOS, thereby inhibiting the conversion of L-arginine to NO. This inhibition can lead to various pathophysiological conditions, including endothelial dysfunction, hypertension, and atherosclerosis. The relationship between ADMA levels and cardiovascular risk has been well documented:
- Inhibition of Nitric Oxide Production : Elevated ADMA levels are associated with reduced NO synthesis, contributing to impaired endothelium-dependent vasodilation. Studies have shown that ADMA concentrations ranging from 3–15 μmol/L can significantly inhibit NO production in vascular tissues .
- Vasoconstriction Effects : When infused intra-arterially, ADMA causes local vasoconstriction, further supporting its role in regulating vascular tone .
Clinical Implications
ADMA has emerged as an important biomarker for cardiovascular risk:
- Cardiovascular Disease : Increased plasma levels of ADMA have been observed in patients with conditions such as hypercholesterolemia, chronic renal failure, and heart failure. These elevated levels correlate with adverse cardiovascular outcomes .
- Therapeutic Target : Given its role in cardiovascular pathology, ADMA is being explored as a target for therapeutic interventions. Strategies such as L-arginine supplementation have shown promise in improving endothelial function in individuals with high ADMA levels .
Data Table: Biological Activity and Implications of this compound
Biological Activity | Mechanism | Clinical Implications |
---|---|---|
Inhibits NOS | Competes with L-arginine for NOS binding | Associated with endothelial dysfunction |
Induces Vasoconstriction | Local infusion leads to reduced vessel diameter | Contributes to hypertension |
Biomarker for Disease | Elevated levels linked to cardiovascular diseases | Potential target for pharmacotherapy |
Case Studies
- End-stage Renal Disease : A study highlighted that patients with end-stage renal disease exhibited significantly higher plasma levels of ADMA compared to healthy controls. This elevation was linked to impaired NO production and increased vascular resistance, underscoring the importance of ADMA in renal pathology .
- Atherosclerosis : Research has demonstrated that ADMA levels are predictive of atherosclerotic progression. In animal models, lowering ADMA resulted in reduced atherosclerotic lesions and improved vascular function, suggesting that modulation of ADMA could be beneficial in preventing cardiovascular events .
- Hypertension : In hypertensive patients, elevated ADMA levels were correlated with decreased endothelial function and increased arterial stiffness. Interventions aimed at reducing ADMA have shown potential in reversing some aspects of vascular dysfunction associated with hypertension .
Research Findings
Recent studies have explored various aspects of ADMA's biological activity:
- DDAH Enzymes : The enzyme dimethylarginine dimethylaminohydrolase (DDAH) plays a critical role in metabolizing ADMA. Research indicates that DDAH1 effectively hydrolyzes ADMA to citrulline and dimethylamine, thus regulating its levels and mitigating its inhibitory effects on NOS .
- Epidemiological Studies : Large-scale studies have identified ADMA as an independent risk factor for cardiovascular mortality across diverse populations. These findings reinforce the need for further investigation into therapeutic strategies targeting ADMA metabolism and action .
Properties
IUPAC Name |
(2S)-2-amino-5-[[amino(dimethylamino)methylidene]amino]pentanoic acid | |
---|---|---|
Source | PubChem | |
URL | https://pubchem.ncbi.nlm.nih.gov | |
Description | Data deposited in or computed by PubChem | |
InChI |
InChI=1S/C8H18N4O2/c1-12(2)8(10)11-5-3-4-6(9)7(13)14/h6H,3-5,9H2,1-2H3,(H2,10,11)(H,13,14)/t6-/m0/s1 | |
Source | PubChem | |
URL | https://pubchem.ncbi.nlm.nih.gov | |
Description | Data deposited in or computed by PubChem | |
InChI Key |
YDGMGEXADBMOMJ-LURJTMIESA-N | |
Source | PubChem | |
URL | https://pubchem.ncbi.nlm.nih.gov | |
Description | Data deposited in or computed by PubChem | |
Canonical SMILES |
CN(C)C(=NCCCC(C(=O)O)N)N | |
Source | PubChem | |
URL | https://pubchem.ncbi.nlm.nih.gov | |
Description | Data deposited in or computed by PubChem | |
Isomeric SMILES |
CN(C)C(=NCCC[C@@H](C(=O)O)N)N | |
Source | PubChem | |
URL | https://pubchem.ncbi.nlm.nih.gov | |
Description | Data deposited in or computed by PubChem | |
Molecular Formula |
C8H18N4O2 | |
Source | PubChem | |
URL | https://pubchem.ncbi.nlm.nih.gov | |
Description | Data deposited in or computed by PubChem | |
DSSTOX Substance ID |
DTXSID401017725 | |
Record name | Dimethyl-L-arginine | |
Source | EPA DSSTox | |
URL | https://comptox.epa.gov/dashboard/DTXSID401017725 | |
Description | DSSTox provides a high quality public chemistry resource for supporting improved predictive toxicology. | |
Molecular Weight |
202.25 g/mol | |
Source | PubChem | |
URL | https://pubchem.ncbi.nlm.nih.gov | |
Description | Data deposited in or computed by PubChem | |
Physical Description |
Solid | |
Record name | Asymmetric dimethylarginine | |
Source | Human Metabolome Database (HMDB) | |
URL | http://www.hmdb.ca/metabolites/HMDB0001539 | |
Description | The Human Metabolome Database (HMDB) is a freely available electronic database containing detailed information about small molecule metabolites found in the human body. | |
Explanation | HMDB is offered to the public as a freely available resource. Use and re-distribution of the data, in whole or in part, for commercial purposes requires explicit permission of the authors and explicit acknowledgment of the source material (HMDB) and the original publication (see the HMDB citing page). We ask that users who download significant portions of the database cite the HMDB paper in any resulting publications. | |
CAS No. |
30315-93-6 | |
Record name | Asymmetric dimethylarginine | |
Source | CAS Common Chemistry | |
URL | https://commonchemistry.cas.org/detail?cas_rn=30315-93-6 | |
Description | CAS Common Chemistry is an open community resource for accessing chemical information. Nearly 500,000 chemical substances from CAS REGISTRY cover areas of community interest, including common and frequently regulated chemicals, and those relevant to high school and undergraduate chemistry classes. This chemical information, curated by our expert scientists, is provided in alignment with our mission as a division of the American Chemical Society. | |
Explanation | The data from CAS Common Chemistry is provided under a CC-BY-NC 4.0 license, unless otherwise stated. | |
Record name | N,N-Dimethylarginine | |
Source | ChemIDplus | |
URL | https://pubchem.ncbi.nlm.nih.gov/substance/?source=chemidplus&sourceid=0030315936 | |
Description | ChemIDplus is a free, web search system that provides access to the structure and nomenclature authority files used for the identification of chemical substances cited in National Library of Medicine (NLM) databases, including the TOXNET system. | |
Record name | N,N-dimethylarginine | |
Source | DrugBank | |
URL | https://www.drugbank.ca/drugs/DB01686 | |
Description | The DrugBank database is a unique bioinformatics and cheminformatics resource that combines detailed drug (i.e. chemical, pharmacological and pharmaceutical) data with comprehensive drug target (i.e. sequence, structure, and pathway) information. | |
Explanation | Creative Common's Attribution-NonCommercial 4.0 International License (http://creativecommons.org/licenses/by-nc/4.0/legalcode) | |
Record name | Dimethyl-L-arginine | |
Source | EPA DSSTox | |
URL | https://comptox.epa.gov/dashboard/DTXSID401017725 | |
Description | DSSTox provides a high quality public chemistry resource for supporting improved predictive toxicology. | |
Record name | N,N-DIMETHYLARGININE | |
Source | FDA Global Substance Registration System (GSRS) | |
URL | https://gsrs.ncats.nih.gov/ginas/app/beta/substances/63CV1GEK3Y | |
Description | The FDA Global Substance Registration System (GSRS) enables the efficient and accurate exchange of information on what substances are in regulated products. Instead of relying on names, which vary across regulatory domains, countries, and regions, the GSRS knowledge base makes it possible for substances to be defined by standardized, scientific descriptions. | |
Explanation | Unless otherwise noted, the contents of the FDA website (www.fda.gov), both text and graphics, are not copyrighted. They are in the public domain and may be republished, reprinted and otherwise used freely by anyone without the need to obtain permission from FDA. Credit to the U.S. Food and Drug Administration as the source is appreciated but not required. | |
Record name | Asymmetric dimethylarginine | |
Source | Human Metabolome Database (HMDB) | |
URL | http://www.hmdb.ca/metabolites/HMDB0001539 | |
Description | The Human Metabolome Database (HMDB) is a freely available electronic database containing detailed information about small molecule metabolites found in the human body. | |
Explanation | HMDB is offered to the public as a freely available resource. Use and re-distribution of the data, in whole or in part, for commercial purposes requires explicit permission of the authors and explicit acknowledgment of the source material (HMDB) and the original publication (see the HMDB citing page). We ask that users who download significant portions of the database cite the HMDB paper in any resulting publications. | |
Melting Point |
195 - 197 °C | |
Record name | Asymmetric dimethylarginine | |
Source | Human Metabolome Database (HMDB) | |
URL | http://www.hmdb.ca/metabolites/HMDB0001539 | |
Description | The Human Metabolome Database (HMDB) is a freely available electronic database containing detailed information about small molecule metabolites found in the human body. | |
Explanation | HMDB is offered to the public as a freely available resource. Use and re-distribution of the data, in whole or in part, for commercial purposes requires explicit permission of the authors and explicit acknowledgment of the source material (HMDB) and the original publication (see the HMDB citing page). We ask that users who download significant portions of the database cite the HMDB paper in any resulting publications. | |
Retrosynthesis Analysis
AI-Powered Synthesis Planning: Our tool employs the Template_relevance Pistachio, Template_relevance Bkms_metabolic, Template_relevance Pistachio_ringbreaker, Template_relevance Reaxys, Template_relevance Reaxys_biocatalysis model, leveraging a vast database of chemical reactions to predict feasible synthetic routes.
One-Step Synthesis Focus: Specifically designed for one-step synthesis, it provides concise and direct routes for your target compounds, streamlining the synthesis process.
Accurate Predictions: Utilizing the extensive PISTACHIO, BKMS_METABOLIC, PISTACHIO_RINGBREAKER, REAXYS, REAXYS_BIOCATALYSIS database, our tool offers high-accuracy predictions, reflecting the latest in chemical research and data.
Strategy Settings
Precursor scoring | Relevance Heuristic |
---|---|
Min. plausibility | 0.01 |
Model | Template_relevance |
Template Set | Pistachio/Bkms_metabolic/Pistachio_ringbreaker/Reaxys/Reaxys_biocatalysis |
Top-N result to add to graph | 6 |
Feasible Synthetic Routes
A: ADMA is an endogenous inhibitor of nitric oxide synthase (NOS) enzymes [, , ]. It competes with L-arginine, the natural substrate of NOS, for binding to the enzyme's active site []. This competitive inhibition reduces the production of nitric oxide (NO), a potent vasodilator, leading to vasoconstriction and potential endothelial dysfunction [, , ].
ANone: Molecular Formula: C6H15N4O2+ * Molecular Weight:* 175.21 g/mol
A: DDAH is an enzyme that specifically metabolizes asymmetric methylarginines, including ADMA, to citrulline [, ]. This catalytic activity regulates ADMA levels and consequently influences NO bioavailability [, ]. There are two isoforms of this enzyme, DDAH1 and DDAH2. Research suggests that single nucleotide polymorphisms (SNPs) in the DDAH1 gene may be associated with pulmonary hypertension risk in patients with bronchopulmonary dysplasia [, ].
A: Research suggests that pentadecanoic acid, 5'-methylthioadenosine (5'-MTA), ADMA, and glutamine may serve as potential biomarkers for diabetic sarcopenia []. These findings provide new insights into the pathophysiology of the disease and could potentially contribute to the development of therapeutic interventions.
A: The presence of the bacterium Eggerthella lenta in the gut microbiome of gnotobiotic mice was associated with decreased levels of ADMA in colon content []. This suggests a potential role of specific gut microbes in modulating ADMA levels and potentially influencing host health.
A: Research using a rat model suggests that chronic treatment with monoamine reuptake inhibitors (MARIs) modulates the expression of dimethylarginine dimethylaminohydrolase-1 (DDAH-1) in the hippocampus []. This modulation is part of wider proteome changes associated with potential long-term functional adaptations in the brain linked to antidepressant activity [].
A: Aberrant histone methylation, including methylation of arginine residues, has been linked to human diseases like cancer []. Understanding the enzymes and mechanisms involved in histone methylation, including the role of ADMA, is crucial for developing targeted therapies.
A: There is no direct connection between ADMA and fungal bioluminescence described in the provided research. Fungal bioluminescence involves a completely different set of molecules and mechanisms, primarily centered around the luciferin 3-hydroxyhispidin [].
ANone: The provided research does not offer specific details about the stability of ADMA under different conditions. Investigating the stability of ADMA in various biological matrices and storage conditions would be essential for research and potential clinical applications.
A: While not explicitly described, researchers likely employ techniques like high-performance liquid chromatography (HPLC) and mass spectrometry (MS) to measure and characterize ADMA in biological samples [, , ].
Disclaimer and Information on In-Vitro Research Products
Please be aware that all articles and product information presented on BenchChem are intended solely for informational purposes. The products available for purchase on BenchChem are specifically designed for in-vitro studies, which are conducted outside of living organisms. In-vitro studies, derived from the Latin term "in glass," involve experiments performed in controlled laboratory settings using cells or tissues. It is important to note that these products are not categorized as medicines or drugs, and they have not received approval from the FDA for the prevention, treatment, or cure of any medical condition, ailment, or disease. We must emphasize that any form of bodily introduction of these products into humans or animals is strictly prohibited by law. It is essential to adhere to these guidelines to ensure compliance with legal and ethical standards in research and experimentation.