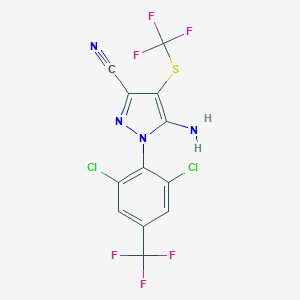
Fipronil-sulfide
Overview
Description
Fipronil-sulfide is a metabolite of the broad-spectrum phenylpyrazole insecticide fipronil. Fipronil itself is widely used in agriculture to control a variety of insect pests. This compound is formed through the reduction of fipronil and is known for its increased toxicity compared to the parent compound .
Mechanism of Action
Target of Action
Fipronil-sulfide, like its parent compound fipronil, primarily targets the γ-aminobutryic acid (GABA) receptors in the central nervous system of invertebrates . These receptors play a crucial role in inhibitory neurotransmission .
Mode of Action
This compound operates by blocking the GABA receptor site on GABA-gated chloride channels . This action disrupts neural transmission, leading to continuous stimulation of neurons and ultimately resulting in the death of target invertebrates .
Biochemical Pathways
The metabolic reactions of fipronil degradation, which lead to the formation of this compound, appear to be the same in different bacteria and are mainly oxidation, reduction, photolysis, and hydrolysis . The enzymes responsible for the degradation are somewhat different across bacterial strains, with the ligninolytic enzyme MnP, the cytochrome P450 enzyme, and esterase playing key roles .
Pharmacokinetics
Fipronil is slightly soluble in water and more soluble in lipids, oils, proteins, and lipophilic organic solvents . This lipophilic nature contributes to its persistence in the environment and its bioaccumulation in organisms . In mammals, fipronil can be metabolized at its trifluoromethylsulfinyl or cyano moieties through three major pathways: oxidation at the sulfinyl moiety to form fipronil-sulfone; reduction at the sulfinyl moiety yielding this compound; and by hydrolysis of the cyano moiety to form fipronil-amide .
Result of Action
The action of this compound, like fipronil, has been found to have significant effects on the growth, development, and reproduction of organisms . It’s worth noting that one of the characteristics of fluorinated compounds like this compound is the production of metabolites more toxic than the initial compound .
Action Environment
This compound, being a degradation product of fipronil, shares its environmental persistence and potential for bioaccumulation . It can be quite persistent in soil systems but is less so in aquatic and sediment systems . The degradation of fipronil and its metabolites, including this compound, can be influenced by various environmental factors . For instance, biodegradation, deemed to be the most effective and environmentally friendly method for the removal of fipronil, can be influenced by factors such as temperature, pH, and the presence of certain microbial species .
Biochemical Analysis
Biochemical Properties
Fipronil-sulfide interacts with several enzymes and proteins. The metabolic reactions of this compound degradation appear to be the same in different bacteria and are mainly oxidation, reduction, photolysis, and hydrolysis . The enzymes and genes responsible for the degradation are somewhat different. The ligninolytic enzyme MnP, the cytochrome P450 enzyme, and esterase play key roles in different strains of bacteria and fungi .
Cellular Effects
This compound has been found to have significant effects on various types of cells and cellular processes . It influences cell function, including any impact on cell signaling pathways, gene expression, and cellular metabolism .
Molecular Mechanism
This compound exerts its effects at the molecular level, including any binding interactions with biomolecules, enzyme inhibition or activation, and changes in gene expression .
Temporal Effects in Laboratory Settings
In laboratory settings, the effects of this compound change over time. This includes information on the product’s stability, degradation, and any long-term effects on cellular function observed in in vitro or in vivo studies .
Dosage Effects in Animal Models
The effects of this compound vary with different dosages in animal models . This includes any threshold effects observed in these studies, as well as any toxic or adverse effects at high doses .
Metabolic Pathways
This compound is involved in several metabolic pathways, including any enzymes or cofactors that it interacts with . This also includes any effects on metabolic flux or metabolite levels .
Transport and Distribution
This compound is transported and distributed within cells and tissues . This includes any transporters or binding proteins that it interacts with, as well as any effects on its localization or accumulation .
Subcellular Localization
This could include any targeting signals or post-translational modifications that direct it to specific compartments or organelles .
Preparation Methods
Synthetic Routes and Reaction Conditions: Fipronil-sulfide can be synthesized through the reduction of fipronil. One common method involves the use of reducing agents such as sodium borohydride (NaBH4) in an appropriate solvent like methanol or ethanol. The reaction is typically carried out at room temperature under an inert atmosphere to prevent oxidation .
Industrial Production Methods: Industrial production of this compound follows similar synthetic routes but on a larger scale. The process involves the use of large reactors and precise control of reaction conditions to ensure high yield and purity. The reduction reaction is monitored using techniques like gas chromatography to ensure complete conversion of fipronil to this compound .
Types of Reactions:
Oxidation: this compound can undergo oxidation to form fipronil-sulfone, another metabolite of fipronil.
Reduction: As mentioned, this compound is formed through the reduction of fipronil.
Photolysis: this compound can also undergo photolytic degradation under UV light.
Common Reagents and Conditions:
Oxidation: Common oxidizing agents include hydrogen peroxide (H2O2) and potassium permanganate (KMnO4).
Reduction: Sodium borohydride (NaBH4) is commonly used as a reducing agent.
Photolysis: UV light is used for photolytic reactions.
Major Products Formed:
Oxidation: Fipronil-sulfone
Reduction: this compound (from fipronil)
Photolysis: Various degradation products including fipronil-desulfinyl.
Scientific Research Applications
Fipronil-sulfide has several applications in scientific research:
Comparison with Similar Compounds
Fipronil: The parent compound, widely used as an insecticide.
Fipronil-sulfone: An oxidation product of fipronil, known for its high toxicity.
Fipronil-desulfinyl: A photolytic degradation product of fipronil.
Uniqueness: Fipronil-sulfide is unique due to its formation through reduction and its increased toxicity compared to fipronil. It is more stable in the environment and poses a higher risk to non-target organisms .
By understanding the properties, preparation methods, chemical reactions, and applications of this compound, researchers can better assess its environmental impact and develop strategies to mitigate its adverse effects.
Biological Activity
Fipronil-sulfide, a degradation product of the widely used insecticide fipronil, has garnered attention due to its biological activity and potential environmental impacts. This article explores the biological effects, degradation pathways, and toxicological implications of this compound based on various studies.
Overview of Fipronil and Its Degradation
Fipronil is a phenylpyrazole insecticide effective against a broad range of pests. However, its persistence in the environment raises concerns about its degradation products, particularly this compound and fipronil sulfone. These metabolites are formed through microbial degradation processes and have been shown to exhibit significant biological activity.
Biological Activity
Toxicological Effects:
Research indicates that this compound can induce toxic effects in non-target organisms. A study involving zebrafish demonstrated that maternal exposure to fipronil resulted in transgenerational toxic effects on offspring, including reduced hatching rates and increased mortality. The presence of this compound in the offspring was noted, suggesting maternal transmission of toxins as a key mechanism of toxicity .
Endocrine Disruption:
this compound has been linked to endocrine disruption, particularly affecting thyroid hormone levels critical for development. The study highlighted that both direct exposure to fipronil and indirect effects through maternal thyroid hormone disruption contributed to the observed toxic effects in offspring .
Degradation Pathways
Fipronil undergoes microbial degradation in various environments, leading to the formation of several metabolites including this compound. Studies have identified bacterial species capable of degrading fipronil effectively. For instance, Pseudomonas and Rhodococcus species have shown high removal efficiencies (over 80%) for fipronil under laboratory conditions .
Metabolite Formation:
The degradation process yields several metabolites, with this compound being one of the major products. The metabolic pathway includes:
- Hydrolysis
- Oxidation
- Reduction processes leading to the formation of this compound and other derivatives .
Case Studies and Research Findings
- Microbial Degradation Study:
-
Transgenerational Toxicity in Zebrafish:
- Study Design: Adult female zebrafish were exposed to environmentally relevant levels of fipronil for 28 days.
- Results: Offspring exhibited a 30% reduction in hatch rates and over double the mortality rate compared to controls. This compound was detected in the offspring, indicating maternal transfer .
- Impact of Fertilizers on Degradation:
Data Summary Table
Study Focus | Key Findings |
---|---|
Microbial Degradation | High efficiency by Pseudomonas and Rhodococcus (85.97%) |
Transgenerational Toxicity | 30% reduction in hatch rates; increased mortality in offspring |
Fertilizer Impact | Reduced total pesticide but not metabolite concentrations |
Properties
IUPAC Name |
5-amino-1-[2,6-dichloro-4-(trifluoromethyl)phenyl]-4-(trifluoromethylsulfanyl)pyrazole-3-carbonitrile | |
---|---|---|
Source | PubChem | |
URL | https://pubchem.ncbi.nlm.nih.gov | |
Description | Data deposited in or computed by PubChem | |
InChI |
InChI=1S/C12H4Cl2F6N4S/c13-5-1-4(11(15,16)17)2-6(14)8(5)24-10(22)9(7(3-21)23-24)25-12(18,19)20/h1-2H,22H2 | |
Source | PubChem | |
URL | https://pubchem.ncbi.nlm.nih.gov | |
Description | Data deposited in or computed by PubChem | |
InChI Key |
FQXWEKADCSXYOC-UHFFFAOYSA-N | |
Source | PubChem | |
URL | https://pubchem.ncbi.nlm.nih.gov | |
Description | Data deposited in or computed by PubChem | |
Canonical SMILES |
C1=C(C=C(C(=C1Cl)N2C(=C(C(=N2)C#N)SC(F)(F)F)N)Cl)C(F)(F)F | |
Source | PubChem | |
URL | https://pubchem.ncbi.nlm.nih.gov | |
Description | Data deposited in or computed by PubChem | |
Molecular Formula |
C12H4Cl2F6N4S | |
Source | PubChem | |
URL | https://pubchem.ncbi.nlm.nih.gov | |
Description | Data deposited in or computed by PubChem | |
DSSTOX Substance ID |
DTXSID50869644 | |
Record name | Fipronil sulfide | |
Source | EPA DSSTox | |
URL | https://comptox.epa.gov/dashboard/DTXSID50869644 | |
Description | DSSTox provides a high quality public chemistry resource for supporting improved predictive toxicology. | |
Molecular Weight |
421.1 g/mol | |
Source | PubChem | |
URL | https://pubchem.ncbi.nlm.nih.gov | |
Description | Data deposited in or computed by PubChem | |
CAS No. |
120067-83-6 | |
Record name | Fipronil sulfide | |
Source | CAS Common Chemistry | |
URL | https://commonchemistry.cas.org/detail?cas_rn=120067-83-6 | |
Description | CAS Common Chemistry is an open community resource for accessing chemical information. Nearly 500,000 chemical substances from CAS REGISTRY cover areas of community interest, including common and frequently regulated chemicals, and those relevant to high school and undergraduate chemistry classes. This chemical information, curated by our expert scientists, is provided in alignment with our mission as a division of the American Chemical Society. | |
Explanation | The data from CAS Common Chemistry is provided under a CC-BY-NC 4.0 license, unless otherwise stated. | |
Record name | 1H-Pyrazole-3-carbonitrile, 5-amino-1-(2,6-dichloro-4-(trifluoromethyl)phenyl)-4-((trifluoromethyl)thio)- | |
Source | ChemIDplus | |
URL | https://pubchem.ncbi.nlm.nih.gov/substance/?source=chemidplus&sourceid=0120067836 | |
Description | ChemIDplus is a free, web search system that provides access to the structure and nomenclature authority files used for the identification of chemical substances cited in National Library of Medicine (NLM) databases, including the TOXNET system. | |
Record name | Fipronil sulfide | |
Source | EPA DSSTox | |
URL | https://comptox.epa.gov/dashboard/DTXSID50869644 | |
Description | DSSTox provides a high quality public chemistry resource for supporting improved predictive toxicology. | |
Record name | 1H-Pyrazole-3-carbonitrile, 5-amino-1-[2,6-dichloro-4-(trifluoromethyl)phenyl]-4-[(trifluoromethyl)thio] | |
Source | European Chemicals Agency (ECHA) | |
URL | https://echa.europa.eu/substance-information/-/substanceinfo/100.107.023 | |
Description | The European Chemicals Agency (ECHA) is an agency of the European Union which is the driving force among regulatory authorities in implementing the EU's groundbreaking chemicals legislation for the benefit of human health and the environment as well as for innovation and competitiveness. | |
Explanation | Use of the information, documents and data from the ECHA website is subject to the terms and conditions of this Legal Notice, and subject to other binding limitations provided for under applicable law, the information, documents and data made available on the ECHA website may be reproduced, distributed and/or used, totally or in part, for non-commercial purposes provided that ECHA is acknowledged as the source: "Source: European Chemicals Agency, http://echa.europa.eu/". Such acknowledgement must be included in each copy of the material. ECHA permits and encourages organisations and individuals to create links to the ECHA website under the following cumulative conditions: Links can only be made to webpages that provide a link to the Legal Notice page. | |
Synthesis routes and methods I
Procedure details
Synthesis routes and methods II
Procedure details
Retrosynthesis Analysis
AI-Powered Synthesis Planning: Our tool employs the Template_relevance Pistachio, Template_relevance Bkms_metabolic, Template_relevance Pistachio_ringbreaker, Template_relevance Reaxys, Template_relevance Reaxys_biocatalysis model, leveraging a vast database of chemical reactions to predict feasible synthetic routes.
One-Step Synthesis Focus: Specifically designed for one-step synthesis, it provides concise and direct routes for your target compounds, streamlining the synthesis process.
Accurate Predictions: Utilizing the extensive PISTACHIO, BKMS_METABOLIC, PISTACHIO_RINGBREAKER, REAXYS, REAXYS_BIOCATALYSIS database, our tool offers high-accuracy predictions, reflecting the latest in chemical research and data.
Strategy Settings
Precursor scoring | Relevance Heuristic |
---|---|
Min. plausibility | 0.01 |
Model | Template_relevance |
Template Set | Pistachio/Bkms_metabolic/Pistachio_ringbreaker/Reaxys/Reaxys_biocatalysis |
Top-N result to add to graph | 6 |
Feasible Synthetic Routes
Q1: How does fipronil-sulfide exert its insecticidal effect?
A1: Like its parent compound, this compound acts as a noncompetitive inhibitor of the γ-aminobutyric acid (GABA) receptors in insects, disrupting nerve signal transmission and ultimately leading to paralysis and death [, ].
Q2: Does this compound affect non-target organisms?
A2: Yes, this compound exhibits toxicity to various non-target organisms, particularly aquatic invertebrates like Chironomus tentans and Hyalella azteca [, ]. It can negatively impact their growth, development, and survival, potentially disrupting aquatic ecosystems.
Q3: What is the molecular formula and weight of this compound?
A3: this compound has the molecular formula C12H4Cl2F6N4S2 and a molecular weight of 437.21 g/mol.
Q4: How persistent is this compound in the environment?
A4: this compound exhibits enhanced persistence in sediments compared to fipronil, particularly under anaerobic conditions [, ]. Estimated half-lives in anaerobic sediments ranged from 195 to over 365 days, indicating long-term persistence and potential for bioaccumulation.
Q5: Does this compound degrade in water?
A5: this compound persists in water, although its persistence is lower than in sediment. Studies show that its half-life in water varies from 21.8 to 35770 days, influenced by factors like pH. The degradation rate decreases with decreasing pH, being slowest at pH 4 [].
Q6: Does this compound participate in any catalytic reactions?
A6: Current research primarily focuses on this compound's presence as a degradation product of fipronil and its toxicological implications. There is limited information regarding its catalytic properties or applications.
Q7: Are there any computational studies exploring this compound interactions?
A7: Yes, recent molecular dynamics simulations investigated the binding of this compound to gut microbiome proteins like Diaminopimelate epimerase (DapF) and Tyrosine Decarboxylase (TDC) []. These studies suggest that this compound binding could potentially inhibit bacterial function and impact neurotransmitter production, warranting further research on its implications for diseases like Parkinson's Disease.
Q8: How does the sulfide group in this compound influence its activity compared to fipronil?
A8: While both compounds target GABA receptors, the sulfide group in this compound can influence its binding affinity and potentially alter its toxicological profile compared to fipronil. Further research is needed to fully elucidate the structure-activity relationships of fipronil and its metabolites.
Q9: What are the formulation strategies for this compound analysis?
A9: Studies utilized various extraction and clean-up methods for this compound analysis in different matrices like soil, eggs, and water [, , , ]. Techniques like solid-phase extraction (SPE), QuEChERS, and SinChERS, coupled with analytical methods like LC-MS/MS and GC-MS, are commonly employed for its detection and quantification.
Q10: What is known about the ADME of this compound?
A10: Research primarily focuses on the environmental fate and toxicity of this compound. Detailed information regarding its absorption, distribution, metabolism, and excretion in specific organisms requires further investigation.
Q11: Are there specific cell-based assays or animal models used to study this compound toxicity?
A11: Studies employed organisms like the earthworm Eisenia foetida, aquatic invertebrates Chironomus tentans and Hyalella azteca, and zebrafish to investigate the bioaccumulation and toxicological effects of this compound [, , ]. These models help assess its potential risks to different species and trophic levels.
Q12: What are the known toxicological effects of this compound?
A12: this compound, like fipronil, primarily affects the nervous system by inhibiting GABA receptors. Research indicates potential adverse effects on aquatic organisms, impacting their growth, development, and survival [, ]. Further research is needed to fully understand its long-term effects and potential risks to human health.
Q13: What are the environmental concerns associated with this compound?
A13: Its persistence in sediment and water raises concerns about its potential for bioaccumulation and biomagnification in the food chain, potentially impacting higher trophic levels []. Its presence in urban waterways emphasizes the need for monitoring and mitigation strategies to minimize its ecological impact [].
Q14: What are the common analytical techniques used for this compound detection and quantification?
A14: Several methods are employed for this compound analysis, including:
- Gas chromatography-mass spectrometry (GC-MS): Provides high sensitivity and selectivity for identifying and quantifying this compound in various matrices [, , , , , ].
- Liquid chromatography-tandem mass spectrometry (LC-MS/MS): Offers rapid and sensitive detection with the ability to analyze multiple compounds simultaneously, making it suitable for multi-residue analysis in complex matrices like food and environmental samples [, , , , , ].
- Ultra-performance liquid chromatography-tandem mass spectrometry (UPLC-MS/MS): Offers enhanced sensitivity and resolution compared to conventional LC-MS/MS, enabling the detection of trace levels of this compound in complex matrices [, ].
- High-performance liquid chromatography (HPLC): Coupled with various detectors, HPLC is used to separate and quantify this compound in different samples [].
Q15: How are analytical methods for this compound validated?
A15: Analytical methods for this compound undergo rigorous validation procedures to ensure accuracy, precision, specificity, linearity, and sensitivity [, ]. These procedures involve analyzing spiked samples at different concentrations, evaluating recovery rates, and determining the limit of detection (LOD) and limit of quantification (LOQ).
Disclaimer and Information on In-Vitro Research Products
Please be aware that all articles and product information presented on BenchChem are intended solely for informational purposes. The products available for purchase on BenchChem are specifically designed for in-vitro studies, which are conducted outside of living organisms. In-vitro studies, derived from the Latin term "in glass," involve experiments performed in controlled laboratory settings using cells or tissues. It is important to note that these products are not categorized as medicines or drugs, and they have not received approval from the FDA for the prevention, treatment, or cure of any medical condition, ailment, or disease. We must emphasize that any form of bodily introduction of these products into humans or animals is strictly prohibited by law. It is essential to adhere to these guidelines to ensure compliance with legal and ethical standards in research and experimentation.