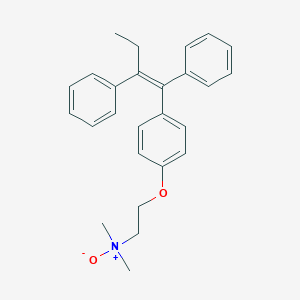
Tamoxifen N-oxide
Overview
Description
Tamoxifen N-oxide is a metabolite of tamoxifen, a well-known selective estrogen receptor modulator used primarily in the treatment of estrogen receptor-positive breast cancer. This compound has garnered interest due to its unique chemical properties and potential biological activities .
Preparation Methods
Synthetic Routes and Reaction Conditions: Tamoxifen N-oxide can be synthesized through the oxidation of tamoxifen. One common method involves the use of flavin-containing monooxygenases (FMOs) which catalyze the formation of this compound from tamoxifen . The reaction typically requires an oxygen source and occurs under mild conditions.
Industrial Production Methods: In an industrial setting, the production of this compound may involve large-scale oxidation processes using optimized conditions to ensure high yield and purity. The use of biocatalysts like FMOs can be scaled up, and continuous flow reactors may be employed to enhance efficiency and control over the reaction parameters.
Chemical Reactions Analysis
Types of Reactions: Tamoxifen N-oxide undergoes various chemical reactions, including:
Oxidation: Further oxidation can lead to the formation of other metabolites.
Reduction: this compound can be reduced back to tamoxifen under specific conditions.
Substitution: It can participate in nucleophilic substitution reactions, where the N-oxide group is replaced by other functional groups.
Common Reagents and Conditions:
Oxidation: Hydrogen peroxide or molecular oxygen in the presence of FMOs.
Reduction: Reducing agents like sodium borohydride or catalytic hydrogenation.
Substitution: Nucleophiles such as amines or thiols under basic conditions.
Major Products Formed:
Oxidation: Further oxidized metabolites.
Reduction: Tamoxifen.
Substitution: Various substituted derivatives depending on the nucleophile used.
Scientific Research Applications
Tamoxifen N-oxide has several applications in scientific research:
Chemistry: Used as a model compound to study oxidation reactions and the role of FMOs.
Biology: Investigated for its potential biological activities, including anti-cancer properties.
Medicine: Explored as a potential therapeutic agent due to its ability to modulate estrogen receptors.
Mechanism of Action
Tamoxifen N-oxide exerts its effects primarily through interaction with estrogen receptors. It binds to these receptors, modulating their activity and influencing the transcription of estrogen-responsive genes. This modulation can inhibit the proliferation of estrogen receptor-positive breast cancer cells. Additionally, this compound may exert effects through non-estrogen receptor pathways, including the induction of oxidative stress and apoptosis in cancer cells .
Comparison with Similar Compounds
Tamoxifen: The parent compound, widely used in breast cancer treatment.
4-Hydroxytamoxifen: A potent metabolite with higher affinity for estrogen receptors.
Endoxifen: Another active metabolite with significant anti-cancer activity.
Comparison: Tamoxifen N-oxide is unique due to its specific oxidation state and the potential for further chemical modifications. Unlike tamoxifen, which is primarily used for its estrogen receptor modulation, this compound’s additional oxidative properties may offer distinct therapeutic advantages and research opportunities .
Biological Activity
Tamoxifen N-oxide is a significant metabolite of tamoxifen, a selective estrogen receptor modulator (SERM) widely used in the treatment of estrogen receptor-positive breast cancer. Understanding the biological activity of this compound is crucial for elucidating its role in the pharmacological effects and potential side effects associated with tamoxifen therapy. This article reviews the metabolic pathways, biological mechanisms, and research findings related to this compound.
Metabolism of Tamoxifen
Tamoxifen undergoes extensive metabolism primarily in the liver, where it is converted into several metabolites, including this compound. The cytochrome P450 enzyme system, particularly CYP2D6, CYP3A4, and CYP2C19, plays a critical role in this metabolic process.
Key Metabolites
- Tamoxifen : The parent compound.
- 4-Hydroxytamoxifen : A potent active metabolite.
- Endoxifen : The most active metabolite, significantly contributing to tamoxifen's therapeutic effects.
- This compound : A less understood metabolite whose biological activity is under investigation.
The metabolic pathway can be summarized as follows:
Metabolite | Formation Enzyme | Biological Activity |
---|---|---|
Tamoxifen | - | Parent compound |
4-Hydroxytamoxifen | CYP2D6 | Active; binds strongly to ER |
Endoxifen | CYP2D6 | Most active; enhances efficacy |
This compound | CYP3A4/CYP2C19 | Less clear; potential storage form |
Estrogen Receptor Modulation
This compound exhibits varying degrees of estrogen receptor (ER) binding affinity compared to its parent compound and other metabolites. While it has been suggested that this compound may not possess significant estrogenic activity, its role in modulating ER activity remains an area of active research. Studies indicate that it may act as a partial agonist or antagonist depending on the tissue context .
Genotoxicity and DNA Interaction
Research has shown that tamoxifen and its metabolites, including this compound, can form DNA adducts, which may lead to genotoxic effects. A study involving DBA/2 mice demonstrated the formation of hepatic DNA adducts following tamoxifen administration, highlighting the potential for this compound to participate in similar interactions . However, evidence suggests that the genotoxicity associated with tamoxifen may not be primarily due to this compound but rather to other metabolites like 4-hydroxytamoxifen.
Pharmacokinetics
Pharmacokinetic studies indicate that the concentration of this compound can vary based on genetic polymorphisms affecting metabolic enzymes. For instance, individuals with certain CYP2D6 genotypes may have altered levels of this metabolite, potentially influencing therapeutic outcomes and toxicity profiles .
Clinical Implications
Several clinical studies have investigated the relationship between tamoxifen metabolism and patient outcomes. For example, a meta-analysis revealed that patients with reduced CYP2D6 activity had poorer survival rates when treated with tamoxifen, suggesting that variations in metabolite levels, including this compound, could impact treatment efficacy .
Toxicity Observations
Reports indicate that higher serum concentrations of tamoxifen and its metabolites correlate with increased toxicity in some patients. This observation underscores the importance of monitoring metabolite levels to optimize therapeutic strategies while minimizing adverse effects .
Properties
IUPAC Name |
2-[4-[(Z)-1,2-diphenylbut-1-enyl]phenoxy]-N,N-dimethylethanamine oxide | |
---|---|---|
Source | PubChem | |
URL | https://pubchem.ncbi.nlm.nih.gov | |
Description | Data deposited in or computed by PubChem | |
InChI |
InChI=1S/C26H29NO2/c1-4-25(21-11-7-5-8-12-21)26(22-13-9-6-10-14-22)23-15-17-24(18-16-23)29-20-19-27(2,3)28/h5-18H,4,19-20H2,1-3H3/b26-25- | |
Source | PubChem | |
URL | https://pubchem.ncbi.nlm.nih.gov | |
Description | Data deposited in or computed by PubChem | |
InChI Key |
YAASNACECBQAFW-QPLCGJKRSA-N | |
Source | PubChem | |
URL | https://pubchem.ncbi.nlm.nih.gov | |
Description | Data deposited in or computed by PubChem | |
Canonical SMILES |
CCC(=C(C1=CC=CC=C1)C2=CC=C(C=C2)OCC[N+](C)(C)[O-])C3=CC=CC=C3 | |
Source | PubChem | |
URL | https://pubchem.ncbi.nlm.nih.gov | |
Description | Data deposited in or computed by PubChem | |
Isomeric SMILES |
CC/C(=C(\C1=CC=CC=C1)/C2=CC=C(C=C2)OCC[N+](C)(C)[O-])/C3=CC=CC=C3 | |
Source | PubChem | |
URL | https://pubchem.ncbi.nlm.nih.gov | |
Description | Data deposited in or computed by PubChem | |
Molecular Formula |
C26H29NO2 | |
Source | PubChem | |
URL | https://pubchem.ncbi.nlm.nih.gov | |
Description | Data deposited in or computed by PubChem | |
DSSTOX Substance ID |
DTXSID101316463 | |
Record name | Tamoxifen N-oxide | |
Source | EPA DSSTox | |
URL | https://comptox.epa.gov/dashboard/DTXSID101316463 | |
Description | DSSTox provides a high quality public chemistry resource for supporting improved predictive toxicology. | |
Molecular Weight |
387.5 g/mol | |
Source | PubChem | |
URL | https://pubchem.ncbi.nlm.nih.gov | |
Description | Data deposited in or computed by PubChem | |
CAS No. |
75504-34-6 | |
Record name | Tamoxifen N-oxide | |
Source | CAS Common Chemistry | |
URL | https://commonchemistry.cas.org/detail?cas_rn=75504-34-6 | |
Description | CAS Common Chemistry is an open community resource for accessing chemical information. Nearly 500,000 chemical substances from CAS REGISTRY cover areas of community interest, including common and frequently regulated chemicals, and those relevant to high school and undergraduate chemistry classes. This chemical information, curated by our expert scientists, is provided in alignment with our mission as a division of the American Chemical Society. | |
Explanation | The data from CAS Common Chemistry is provided under a CC-BY-NC 4.0 license, unless otherwise stated. | |
Record name | Tamoxifen N-oxide | |
Source | ChemIDplus | |
URL | https://pubchem.ncbi.nlm.nih.gov/substance/?source=chemidplus&sourceid=0075504346 | |
Description | ChemIDplus is a free, web search system that provides access to the structure and nomenclature authority files used for the identification of chemical substances cited in National Library of Medicine (NLM) databases, including the TOXNET system. | |
Record name | Tamoxifen N-oxide | |
Source | EPA DSSTox | |
URL | https://comptox.epa.gov/dashboard/DTXSID101316463 | |
Description | DSSTox provides a high quality public chemistry resource for supporting improved predictive toxicology. | |
Retrosynthesis Analysis
AI-Powered Synthesis Planning: Our tool employs the Template_relevance Pistachio, Template_relevance Bkms_metabolic, Template_relevance Pistachio_ringbreaker, Template_relevance Reaxys, Template_relevance Reaxys_biocatalysis model, leveraging a vast database of chemical reactions to predict feasible synthetic routes.
One-Step Synthesis Focus: Specifically designed for one-step synthesis, it provides concise and direct routes for your target compounds, streamlining the synthesis process.
Accurate Predictions: Utilizing the extensive PISTACHIO, BKMS_METABOLIC, PISTACHIO_RINGBREAKER, REAXYS, REAXYS_BIOCATALYSIS database, our tool offers high-accuracy predictions, reflecting the latest in chemical research and data.
Strategy Settings
Precursor scoring | Relevance Heuristic |
---|---|
Min. plausibility | 0.01 |
Model | Template_relevance |
Template Set | Pistachio/Bkms_metabolic/Pistachio_ringbreaker/Reaxys/Reaxys_biocatalysis |
Top-N result to add to graph | 6 |
Feasible Synthetic Routes
Q1: Which enzymes are primarily responsible for the formation of TNO from TAM?
A1: The flavin-containing monooxygenase (FMO) enzymes, particularly FMO1 and FMO3, are the major catalysts for the N-oxidation of TAM to TNO. [, ]
Q2: Are there species-specific differences in the enzymes involved in TNO formation?
A2: Yes, human FMO1 is not expressed in adults, making FMO3 the primary enzyme responsible for TNO formation in humans. []
Q3: Can TNO be converted back to TAM in biological systems?
A3: Yes, several human cytochrome P450 (CYP) enzymes, notably CYP1A1, CYP2A6, and CYP3A4, have been shown to reduce TNO back to TAM. [] Interestingly, even reduced hemoglobin exhibits this reductive capability. []
Q4: Does the reduction of TNO back to TAM impact TAM's therapeutic efficacy?
A4: The potential for a metabolic cycle between TAM and TNO in vivo raises questions about its impact on TAM's overall efficacy and duration of action. This remains an active area of research. []
Q5: What other metabolic pathways are important for TAM besides N-oxidation?
A5: In addition to N-oxidation, TAM undergoes other significant metabolic transformations, including:
- N-demethylation: Primarily catalyzed by CYP3A4, this pathway leads to the formation of N-desmethyltamoxifen. [, ]
- 4-hydroxylation: This pathway produces 4-hydroxytamoxifen, a more potent antiestrogen than TAM itself. [, , ]
- α-hydroxylation: This pathway leads to the formation of α-hydroxytamoxifen, a precursor to reactive metabolites capable of forming DNA adducts. [, , ]
Q6: Are there differences in the metabolic profiles of TAM across different species?
A6: Yes, significant species-related differences exist in TAM metabolism. For instance:
- Mice: Exhibit rapid metabolism of TAM, primarily to TNO, potentially contributing to their lower susceptibility to TAM-induced liver cancer. [, ]
- Rats: Show a greater propensity for forming α-hydroxytamoxifen and its DNA-reactive metabolites compared to humans, potentially explaining their higher susceptibility to TAM-induced liver cancer. [, ]
Q7: Can TNO itself form DNA adducts?
A7: While TNO itself does not appear to directly form DNA adducts, studies have identified α-(N2-deoxyguanosinyl)tamoxifen N-oxide as a DNA adduct in the liver of mice treated with TAM. [] This suggests that TNO might be further metabolized to reactive species capable of DNA modification.
Q8: What other TAM metabolites are known to contribute to DNA adduct formation?
A8: The primary DNA-reactive metabolite of TAM is thought to be an α-sulfooxy metabolite, formed by the enzyme hydroxysteroid sulfotransferase 2A1 (SULT2A1) acting on α-hydroxytamoxifen. [, ]
Q9: What analytical techniques are commonly used to study TAM and its metabolites, including TNO?
A9: A range of analytical techniques is employed for the analysis of TAM and its metabolites, including:
- High-performance liquid chromatography (HPLC): Frequently coupled with ultraviolet (UV) detection or mass spectrometry (MS) for separation and quantification of TAM and its metabolites in various matrices. [, , , , , , ]
- Liquid chromatography-tandem mass spectrometry (LC-MS/MS): Offers high sensitivity and specificity for the identification and quantification of TAM and its metabolites in complex biological samples. [, , , ]
- 32P-Postlabeling/HPLC: This technique is particularly valuable for the detection and quantification of TAM-DNA adducts in tissues. [, , , ]
- Electrochemiluminescence (ECL): ECL-based arrays coupled with liver microsomes have been used to study the relative DNA damage caused by TAM metabolites in different species. []
Q10: What are the challenges associated with the analysis of TNO?
A10: Analyzing TNO presents unique challenges due to:
- Its potential reduction back to TAM during sample preparation and analysis: This necessitates careful optimization of methods to minimize this conversion. []
- The presence of numerous TAM metabolites in biological samples: This requires methods with high selectivity to differentiate TNO from other metabolites. []
Disclaimer and Information on In-Vitro Research Products
Please be aware that all articles and product information presented on BenchChem are intended solely for informational purposes. The products available for purchase on BenchChem are specifically designed for in-vitro studies, which are conducted outside of living organisms. In-vitro studies, derived from the Latin term "in glass," involve experiments performed in controlled laboratory settings using cells or tissues. It is important to note that these products are not categorized as medicines or drugs, and they have not received approval from the FDA for the prevention, treatment, or cure of any medical condition, ailment, or disease. We must emphasize that any form of bodily introduction of these products into humans or animals is strictly prohibited by law. It is essential to adhere to these guidelines to ensure compliance with legal and ethical standards in research and experimentation.