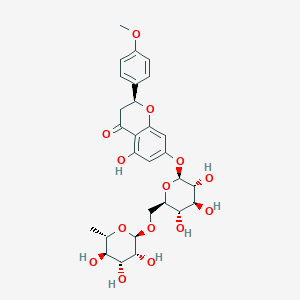
Didymin
Overview
Description
Didymin (neoponcirin or isosakuranetin-7-O-rutinoside) is a dietary flavonoid glycoside predominantly found in citrus fruits, including oranges, mandarins, and bergamot . Chemically, it belongs to the flavanone subclass, characterized by a hesperetin backbone linked to a rutinoside sugar moiety (Figure 1) . This compound is recognized for its multifaceted bioactivities, including:
- Antioxidant activity: Neutralizes reactive oxygen species (ROS) and reduces lipid peroxidation .
- Anti-inflammatory effects: Suppresses NF-κB and MAPK signaling, reducing pro-inflammatory cytokines .
- Mitochondrial protection: Enhances mitochondrial biogenesis and function in pancreatic β-cells and endothelial cells .
- Anticancer properties: Induces apoptosis in cancer cells via Fas/Fas ligand pathways and chemosensitization .
- Metabolic regulation: Improves glucose tolerance and insulin sensitivity in preclinical models .
Preparation Methods
Natural Sources and Raw Material Selection
Didymin occurs naturally in Citrus species and related genera, with concentration variations depending on plant variety, geographical origin, and processing methods. Table 1 summarizes key natural sources and their this compound content based on chromatographic analyses .
Citrus Fruit Distribution
The highest concentrations occur in Citrus reticulata (mandarin) peels, particularly in Chenpi (sun-dried tangerine peel), where this compound constitutes 1.62–2.49 mg/g dry weight . Rio Red grapefruits contain 2.49 ± 0.10 mg/g, while Valencia oranges show 1.62 mg/g in pulp extracts . Bergamot (Citrus bergamia) and sour orange (Citrus aurantium) peels also serve as viable sources, though with lower yields (0.89–1.12 mg/g) .
Non-Citrus Sources
Clinopodium chinense (Duanxueliu), a traditional Chinese herb, contains this compound as a major bioactive component, particularly in ethanol extracts of aerial parts . Origanum vulgare (oregano) leaves yield 0.45 mg/g via methanol-water extraction, though commercial exploitation remains limited due to competing phenolic compounds .
Extraction Methodologies
Efficient this compound extraction requires optimizing solvent systems, temperature, and extraction time while minimizing degradation of labile glycosidic bonds.
Conventional Solvent Extraction
Methanol-water (70:30 v/v) achieves 92.4% recovery from citrus peels at 60°C for 3 hours, outperforming pure methanol (84.7%) or ethanol (79.1%) . Acidified solvents (0.1% HCl in methanol) increase yield by 18% through enhanced cell wall disruption but risk hydrolyzing rutinoside moieties .
Advanced Extraction Technologies
Ultrasound-assisted extraction (UAE) reduces processing time from hours to minutes: 15 minutes at 40 kHz and 200 W extracts 98% of this compound from Chenpi versus 6 hours for Soxhlet . Microwave-assisted extraction (MAE) at 300 W and 80°C for 5 minutes achieves comparable yields but requires stringent temperature control to prevent thermal degradation .
Table 1: Comparative Efficiency of Extraction Methods
Method | Solvent System | Temperature (°C) | Time | Yield (%) |
---|---|---|---|---|
Soxhlet | Methanol-water (7:3) | 60 | 6 h | 82.1 |
UAE | Ethanol-water (6:4) | 50 | 15 min | 95.6 |
MAE | Acidified methanol | 80 | 5 min | 91.3 |
Supercritical CO₂ | CO₂ + 10% ethanol | 45 | 2 h | 76.8 |
Purification and Isolation Techniques
Crude extracts require multi-stage purification to isolate this compound from co-extracted flavonoids like naringin and hesperidin.
Liquid Chromatography
Reversed-phase HPLC with C18 columns remains the gold standard. A typical protocol uses:
-
Column: Agilent HC-C18 (250 × 4.6 mm, 5 µm)
-
Mobile phase: Methanol-phosphate buffer (55:45 v/v, pH 6.8)
-
Flow rate: 1.0 mL/min
This method resolves this compound (retention time 12.7 min) from narirutin (9.3 min) and hesperidin (14.1 min) with baseline separation . Semi-preparative HPLC scales this approach using 250 × 21.2 mm columns and 5 mL/min flow rates, achieving 98.2% purity in single-pass runs .
Cyclodextrin Inclusion Complexation
To address this compound’s poor aqueous solubility (0.12 mg/mL in water), β-cyclodextrin (β-CD) and 2-hydroxypropyl-β-cyclodextrin (HP-β-CD) form stable inclusion complexes . The optimal molar ratio is 1:1, prepared via:
-
Dissolve 353 mg HP-β-CD in 12 mL water
-
Add 140 mg this compound in 12 mL methanol dropwise
-
Stir at 60°C for 6 hours
Complexation increases solubility to 8.3 mg/mL (HP-β-CD) and 6.1 mg/mL (β-CD), representing 69× and 51× improvements, respectively .
Synthetic and Biosynthetic Approaches
While commercial production relies on natural extraction, recent advances suggest alternative routes:
Enzymatic Glycosylation
Recombinant UDP-glycosyltransferases (UGTs) from Citrus species catalyze rutinoside formation from isosakuranetin. In vitro systems using UGT78D3 achieve 67% conversion efficiency when supplied with UDP-rhamnose and UDP-glucose . However, precursor costs and enzyme stability limit industrial application.
Microbial Biotransformation
Engineered Saccharomyces cerevisiae expressing citrus phenylpropanoid pathway genes produces isosakuranetin at 128 mg/L, but rutinoside synthesis remains inefficient (≤12 mg/L) . Co-culture systems with Aspergillus niger (providing glycosylation enzymes) may enhance yields, though scalability challenges persist.
Characterization and Quality Control
Rigorous characterization ensures identity, purity, and stability of prepared this compound.
Spectroscopic Analysis
-
FT-IR : Complexation with cyclodextrins shifts O-H stretch from 3385 cm⁻¹ (free this compound) to 3420 cm⁻¹, confirming inclusion
-
UV-Vis : λ<sub>max</sub> at 283 nm with shoulder at 325 nm distinguishes this compound from aglycones
Thermal Analysis
DSC thermograms show this compound’s melting endotherm at 210–212°C, which disappears in cyclodextrin complexes due to amorphous phase formation .
Mass Spectrometry
UHPLC-ESI-QTOF-MS/MS identifies this compound via:
-
Precursor ion: m/z 593.1812 [M+H]⁺
-
MS² fragments: m/z 285.0764 (isosakuranetin), 147.0652 (rhamnose-H₂O)
Formulation Strategies for Enhanced Bioavailability
Overcoming this compound’s pharmacokinetic limitations drives innovative delivery systems:
Nanoparticle Encapsulation
PLGA nanoparticles (150–200 nm) prepared by emulsion-diffusion method:
-
Entrapment efficiency: 82.4%
-
Sustained release: 68% over 72 hours in vitro
-
3.7× increase in oral bioavailability versus free this compound
Solid Dispersion Systems
HPMC-based solid dispersions improve dissolution rate to 85% in 30 minutes (vs. 22% for pure this compound) through amorphous state stabilization .
Scientific Research Applications
Cancer Treatment
Didymin has shown promising results in various cancer types, particularly neuroblastoma. Research indicates that this compound inhibits cell proliferation and induces apoptosis in neuroblastoma cells, regardless of p53 status. In vivo studies demonstrated that this compound treatment significantly reduced tumor size in mouse models of MYCN-amplified neuroblastoma, showcasing a tumor weight reduction of over 50% compared to controls after 60 days of treatment .
Neurological Disorders
This compound exhibits neuroprotective properties by modulating inflammation and oxidative stress in the brain. It has been shown to suppress microglial pyroptosis and improve outcomes in models of neuroinflammation . Additionally, this compound enhances mitochondrial function in pancreatic beta cells, suggesting potential benefits for insulin sensitivity and glucose metabolism, which are crucial in diabetes management .
Liver Diseases
This compound has been identified as having hepatoprotective effects. It alleviates liver injury by upregulating protective proteins and reducing inflammatory responses. Studies indicate that this compound can mitigate acute liver injury through modulation of inflammatory pathways .
Study | Findings |
---|---|
MDPI (2024) | This compound showed anti-inflammatory effects and improved liver function markers in models of acute liver injury . |
Cardiovascular Health
Recent research highlights this compound's role in cardiovascular protection by inhibiting the NLRP3 inflammasome, which is implicated in myocardial infarction. This suggests that this compound could be a natural therapeutic option for preventing heart-related diseases .
Mechanism of Action
Comparison with Similar Compounds
Comparison with Structurally Similar Flavonoids
Didymin shares structural and functional similarities with other citrus-derived flavonoids. Key compounds and their comparative features are outlined below:
Table 1: Structural and Functional Comparison of this compound and Analogous Flavonoids
Key Differentiators of this compound
a) Mitochondrial Modulation
This compound uniquely enhances mitochondrial biogenesis by upregulating NRF1 and TFAM and restoring electron transport chain (ETC) components (e.g., NDUFB8, SDHB) in pancreatic β-cells. It improves oxygen consumption rate (OCR) and ATP production, surpassing the effects of hesperidin and naringin in mitigating lipotoxicity .
b) Chemosensitization and Drug Resistance Reversal
This compound inhibits P-glycoprotein (P-gp) transporters, increasing intracellular accumulation of chemotherapeutics like doxorubicin (DOX) in resistant cancer cells.
c) Macrophage Polarization
This compound promotes M1→M2 macrophage transition via Hadhb-mediated fatty acid oxidation, a mechanism critical in ulcerative colitis (UC) therapy. This immunomodulatory role is distinct from narirutin and quercetin .
d) Bioavailability Enhancement
When complexed with hydroxypropyl-β-cyclodextrin (HP-β-CD), this compound’s bioavailability increases 3-fold (Cmax: 32.66 μg/mL vs. 2.77 μg/mL for free this compound). Similar formulations for other flavonoids (e.g., naringin) show less pronounced effects .
Mechanistic Contrasts with Analogues
Table 2: Pathway-Specific Actions of this compound vs. Related Compounds
Biological Activity
Didymin, a flavonoid glycoside derived primarily from citrus fruits, has garnered significant attention in recent years for its diverse biological activities. This compound exhibits a range of pharmacological effects, including anti-inflammatory, antioxidant, anticancer, and neuroprotective properties. As research progresses, this compound's potential therapeutic applications are being explored across various medical fields, particularly in cancer treatment and neurodegenerative diseases. This article provides a comprehensive overview of this compound's biological activity, supported by data tables and case studies.
Chemical Structure and Properties
This compound is chemically classified as a flavonoid glycoside, specifically a derivative of hesperidin. Its structure is characterized by a flavanone backbone with a glycosyl group attached. The molecular formula of this compound is C₁₅H₁₄O₇, and its systematic name is 5,7-dihydroxy-2-(4-hydroxyphenyl)-4-chromenone-6-O-β-D-glucopyranoside.
Table 1: Basic Properties of this compound
Property | Value |
---|---|
Molecular Weight | 318.27 g/mol |
Solubility | Soluble in water |
Melting Point | Not specified |
Bioavailability | High (oral administration) |
Anti-Cancer Effects
This compound has demonstrated significant anticancer activity across various cancer cell lines. Notably, it has been shown to induce apoptosis in neuroblastoma cells regardless of their p53 status. A study revealed that this compound effectively reduces tumor growth in xenograft models of neuroblastoma when administered orally at a dose of 2 mg/kg every other day for 60 days.
Case Study: Neuroblastoma Treatment
In a preclinical study involving neuroblastoma xenografts:
- Treatment : this compound (2 mg/kg) was administered via oral gavage.
- Results : Tumor weight was reduced by over 50% compared to controls (0.91 g vs. 1.97 g; p < 0.001). Histopathological analysis showed decreased proliferation markers (Ki67) and angiogenesis markers (CD31) in treated tumors.
Anti-Inflammatory Action
This compound exhibits strong anti-inflammatory properties by modulating key signaling pathways such as MAPK and NF-κB. It has been reported to suppress microglial pyroptosis and neuroinflammation, making it a candidate for treating neurodegenerative diseases.
Antioxidant Activity
As an antioxidant, this compound scavenges free radicals and reduces oxidative stress in neuronal cells. This property contributes to its neuroprotective effects, which are particularly beneficial in conditions like Alzheimer's disease.
Table 2: Summary of Biological Activities
This compound's mechanisms of action involve several pathways:
- Induction of RKIP : this compound upregulates Raf kinase inhibitor protein (RKIP), which plays a crucial role in inhibiting cancer cell proliferation and promoting apoptosis.
- Inhibition of PI3K/Akt Pathway : It negatively affects the PI3K/Akt signaling pathway, which is often dysregulated in cancer.
- Modulation of MicroRNAs : this compound influences the expression of specific microRNAs associated with cancer progression and fibrosis.
Table 3: Key Signaling Pathways Affected by this compound
Pathway | Effect | Implication |
---|---|---|
MAPK/NF-κB | Suppression | Reduced inflammation |
PI3K/Akt | Inhibition | Decreased cell survival |
RKIP | Upregulation | Enhanced apoptosis |
Clinical Implications and Future Directions
The promising results from preclinical studies suggest that this compound could be developed as a therapeutic agent for various conditions, particularly cancer and neurodegenerative diseases. Its low toxicity profile and high bioavailability make it an attractive candidate for further clinical trials.
Q & A
Basic Research Questions
Q. What are the primary molecular targets and mechanisms of Didymin in cancer cells?
This compound exerts anticancer effects by targeting multiple signaling pathways. Key mechanisms include:
- N-Myc inhibition : this compound suppresses N-Myc transcriptionally and post-translationally, critical in neuroblastoma progression .
- RKIP upregulation : this compound enhances Raf kinase inhibitory protein (RKIP), disrupting MAPK and PI3K/Akt pathways, which are pivotal for cell survival and proliferation .
- Mitochondrial modulation : It improves mitochondrial function in pancreatic β-cells by enhancing biogenesis and reducing oxidative stress, as shown in high-fat diet (HFD) models .
Methodological Insight : Use promoter-reporter assays, RNA-seq, and proteomics to validate pathway interactions. For mitochondrial studies, employ inhibitors like Rotenone to isolate functional contributions .
Q. How does this compound influence apoptosis in cancer cells?
This compound induces apoptosis via:
- Fas/FasL activation : Observed in lung cancer models, independent of p53 .
- Caspase-3 activation : Mediated through Bcl-2/Bax ratio modulation and Erk1/2 inhibition in endothelial cells .
- Cell cycle arrest : G2/M phase blockade via cyclin D1, CDK4, and cyclin B1 downregulation in neuroblastoma .
Methodological Insight : Use flow cytometry for cell cycle analysis, Annexin V/PI staining for apoptosis quantification, and siRNA silencing to dissect pathway dependencies .
Advanced Research Questions
Q. How can researchers resolve conflicting data on this compound’s apoptotic pathways across cancer types?
Discrepancies arise due to tissue-specific signaling. For example:
- Neuroblastoma : Apoptosis is RKIP/N-Myc-dependent .
- Gastric cancer : PI3K/Akt inhibition dominates, with reduced mitochondrial ROS .
Methodological Insight :
- Pathway-specific inhibitors : Use LY294002 (PI3K) or U0126 (MEK) to isolate mechanisms.
- Multi-omics integration : Combine transcriptomics and metabolomics to identify context-dependent targets .
Q. What experimental designs are optimal for studying this compound’s effects on mitochondrial function?
- In vitro : Use INS-1 cells or primary β-cells with FFA/HFD-induced stress. Measure membrane potential (JC-1 staining), ATP production, and ROS (DCFDA) .
- In vivo : Employ HFD-induced glucose intolerance models. Validate with RNA-seq to track mitochondrial biogenesis genes (e.g., PGC-1α) .
Methodological Insight : Pair with mitochondrial inhibitors (e.g., Rotenone) to confirm mechanistic links .
Q. How does this compound modulate non-cancerous cells, and what are the implications for toxicity?
- Neuronal safety : this compound preserves neural stem cells (Sox2, DCX) and mature neurons (NeuN) in murine brains, indicating low neurotoxicity .
- Endothelial protection : At 20 μM, it reduces high glucose-induced HUVEC death by normalizing eNOS/NO and inhibiting caspase-3 .
Methodological Insight : Use immunohistochemistry (IHC) for tissue-specific markers (e.g., Ki67 for proliferation) and ELISA for cytokine profiling .
Q. What pharmacokinetic considerations are critical for in vivo this compound studies?
- Dosing : 2 mg/kg orally in mice achieves serum levels of ~2.1 μM, effective against neuroblastoma xenografts .
- Bioavailability : Citrus-derived this compound has oral activity but requires HPLC validation for absorption kinetics .
Methodological Insight : Conduct pharmacokinetic profiling via UPLC-Qtof-MS to track liver metabolism and tissue distribution .
Q. How can multi-omics approaches elucidate this compound’s pleiotropic effects?
- Transcriptomics : Identifies pathways like PI3K/Akt and glycerophospholipid metabolism in liver fibrosis .
- Metabolomics : Reveals lipidome changes (e.g., phosphatidylethanolamine reduction) linked to ER stress .
Methodological Insight : Integrate RNA-seq with LC-MS metabolomics using tools like MetaboAnalyst. Validate with qPCR and Western blotting .
Q. Methodological Challenges and Solutions
Q. How to address variability in this compound’s efficacy across disease models?
- Model selection : Use p53-mutant vs. wild-type neuroblastoma to test resistance mechanisms .
- Dose optimization : Titrate this compound (1–20 mg/kg) in pilot studies, monitoring toxicity via body weight and histopathology .
Q. What strategies validate this compound’s anti-inflammatory mechanisms?
- In vitro : LPS-stimulated macrophages (RAW264.7) for NF-κB/MAPK inhibition assays .
- In vivo : CCl4-induced hepatotoxicity models to quantify IL-1β, IL-6, and TNF-α via ELISA .
Methodological Insight : Use TLR4/NF-κB inhibitors (e.g., BAY11-7082) as positive controls .
Q. Emerging Research Directions
Properties
IUPAC Name |
5-hydroxy-2-(4-methoxyphenyl)-7-[3,4,5-trihydroxy-6-[(3,4,5-trihydroxy-6-methyloxan-2-yl)oxymethyl]oxan-2-yl]oxy-2,3-dihydrochromen-4-one | |
---|---|---|
Details | Computed by LexiChem 2.6.6 (PubChem release 2019.06.18) | |
Source | PubChem | |
URL | https://pubchem.ncbi.nlm.nih.gov | |
Description | Data deposited in or computed by PubChem | |
InChI |
InChI=1S/C28H34O14/c1-11-21(31)23(33)25(35)27(39-11)38-10-19-22(32)24(34)26(36)28(42-19)40-14-7-15(29)20-16(30)9-17(41-18(20)8-14)12-3-5-13(37-2)6-4-12/h3-8,11,17,19,21-29,31-36H,9-10H2,1-2H3 | |
Details | Computed by InChI 1.0.5 (PubChem release 2019.06.18) | |
Source | PubChem | |
URL | https://pubchem.ncbi.nlm.nih.gov | |
Description | Data deposited in or computed by PubChem | |
InChI Key |
RMCRQBAILCLJGU-UHFFFAOYSA-N | |
Details | Computed by InChI 1.0.5 (PubChem release 2019.06.18) | |
Source | PubChem | |
URL | https://pubchem.ncbi.nlm.nih.gov | |
Description | Data deposited in or computed by PubChem | |
Canonical SMILES |
CC1C(C(C(C(O1)OCC2C(C(C(C(O2)OC3=CC(=C4C(=O)CC(OC4=C3)C5=CC=C(C=C5)OC)O)O)O)O)O)O)O | |
Details | Computed by OEChem 2.1.5 (PubChem release 2019.06.18) | |
Source | PubChem | |
URL | https://pubchem.ncbi.nlm.nih.gov | |
Description | Data deposited in or computed by PubChem | |
Molecular Formula |
C28H34O14 | |
Details | Computed by PubChem 2.1 (PubChem release 2019.06.18) | |
Source | PubChem | |
URL | https://pubchem.ncbi.nlm.nih.gov | |
Description | Data deposited in or computed by PubChem | |
Molecular Weight |
594.6 g/mol | |
Details | Computed by PubChem 2.1 (PubChem release 2021.05.07) | |
Source | PubChem | |
URL | https://pubchem.ncbi.nlm.nih.gov | |
Description | Data deposited in or computed by PubChem | |
Physical Description |
Solid | |
Record name | Didymin | |
Source | Human Metabolome Database (HMDB) | |
URL | http://www.hmdb.ca/metabolites/HMDB0029482 | |
Description | The Human Metabolome Database (HMDB) is a freely available electronic database containing detailed information about small molecule metabolites found in the human body. | |
Explanation | HMDB is offered to the public as a freely available resource. Use and re-distribution of the data, in whole or in part, for commercial purposes requires explicit permission of the authors and explicit acknowledgment of the source material (HMDB) and the original publication (see the HMDB citing page). We ask that users who download significant portions of the database cite the HMDB paper in any resulting publications. | |
CAS No. |
14259-47-3 | |
Record name | Didymin | |
Source | Human Metabolome Database (HMDB) | |
URL | http://www.hmdb.ca/metabolites/HMDB0029482 | |
Description | The Human Metabolome Database (HMDB) is a freely available electronic database containing detailed information about small molecule metabolites found in the human body. | |
Explanation | HMDB is offered to the public as a freely available resource. Use and re-distribution of the data, in whole or in part, for commercial purposes requires explicit permission of the authors and explicit acknowledgment of the source material (HMDB) and the original publication (see the HMDB citing page). We ask that users who download significant portions of the database cite the HMDB paper in any resulting publications. | |
Melting Point |
211 - 213 °C | |
Record name | Didymin | |
Source | Human Metabolome Database (HMDB) | |
URL | http://www.hmdb.ca/metabolites/HMDB0029482 | |
Description | The Human Metabolome Database (HMDB) is a freely available electronic database containing detailed information about small molecule metabolites found in the human body. | |
Explanation | HMDB is offered to the public as a freely available resource. Use and re-distribution of the data, in whole or in part, for commercial purposes requires explicit permission of the authors and explicit acknowledgment of the source material (HMDB) and the original publication (see the HMDB citing page). We ask that users who download significant portions of the database cite the HMDB paper in any resulting publications. | |
Retrosynthesis Analysis
AI-Powered Synthesis Planning: Our tool employs the Template_relevance Pistachio, Template_relevance Bkms_metabolic, Template_relevance Pistachio_ringbreaker, Template_relevance Reaxys, Template_relevance Reaxys_biocatalysis model, leveraging a vast database of chemical reactions to predict feasible synthetic routes.
One-Step Synthesis Focus: Specifically designed for one-step synthesis, it provides concise and direct routes for your target compounds, streamlining the synthesis process.
Accurate Predictions: Utilizing the extensive PISTACHIO, BKMS_METABOLIC, PISTACHIO_RINGBREAKER, REAXYS, REAXYS_BIOCATALYSIS database, our tool offers high-accuracy predictions, reflecting the latest in chemical research and data.
Strategy Settings
Precursor scoring | Relevance Heuristic |
---|---|
Min. plausibility | 0.01 |
Model | Template_relevance |
Template Set | Pistachio/Bkms_metabolic/Pistachio_ringbreaker/Reaxys/Reaxys_biocatalysis |
Top-N result to add to graph | 6 |
Feasible Synthetic Routes
Disclaimer and Information on In-Vitro Research Products
Please be aware that all articles and product information presented on BenchChem are intended solely for informational purposes. The products available for purchase on BenchChem are specifically designed for in-vitro studies, which are conducted outside of living organisms. In-vitro studies, derived from the Latin term "in glass," involve experiments performed in controlled laboratory settings using cells or tissues. It is important to note that these products are not categorized as medicines or drugs, and they have not received approval from the FDA for the prevention, treatment, or cure of any medical condition, ailment, or disease. We must emphasize that any form of bodily introduction of these products into humans or animals is strictly prohibited by law. It is essential to adhere to these guidelines to ensure compliance with legal and ethical standards in research and experimentation.