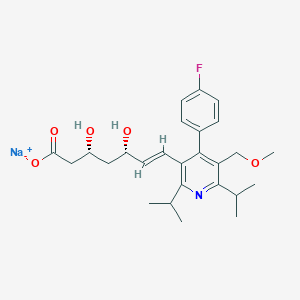
Cerivastatin sodium
Overview
Description
- It was used alongside dietary changes to reduce lipid levels and lower the risk of cardiovascular events.
- Notably, cerivastatin was voluntarily withdrawn from the U.S. market in 2001 due to reports of fatal rhabdomyolysis, a severe adverse reaction .
Cerivastatin sodium: is a synthetic lipid-lowering agent, classified as an HMG-CoA reductase inhibitor (also known as a statin). Its generic name is .
Mechanism of Action
Target of Action
Cerivastatin sodium primarily targets the enzyme 3-hydroxy-3-methylglutaryl-coenzyme A reductase (HMG-CoA reductase) . This enzyme plays a crucial role in the synthesis of cholesterol, a type of lipid that is essential for various cellular functions but can lead to cardiovascular diseases when present in excess .
Mode of Action
this compound acts as a competitive inhibitor of HMG-CoA reductase . It binds to the enzyme and prevents it from converting HMG-CoA to mevalonate, a key step in the biosynthesis of cholesterol . This inhibition leads to a decrease in cholesterol levels within hepatic cells, which in turn triggers an upregulation of LDL-receptors and an increase in hepatic uptake of LDL-cholesterol from the circulation .
Biochemical Pathways
The primary biochemical pathway affected by this compound is the mevalonate pathway , which is responsible for the production of cholesterol . By inhibiting HMG-CoA reductase, this compound disrupts this pathway, leading to reduced cholesterol synthesis . The drug also influences the expression of LDL-receptors, thereby affecting the pathway of LDL-cholesterol uptake into hepatic cells .
Pharmacokinetics
this compound exhibits a mean absolute oral bioavailability of approximately 60% . It is readily and completely absorbed from the gastrointestinal tract, with plasma concentrations peaking 2 to 3 hours post-administration . The drug is highly bound to plasma proteins (>99%), and its volume of distribution at steady state indicates moderate penetration into tissues . This compound is exclusively cleared via metabolism, with no unchanged drug excreted . It undergoes two main oxidative biotransformation reactions, leading to the formation of active metabolites .
Result of Action
The primary result of this compound’s action is a decrease in cholesterol levels in hepatic cells . This reduction in intracellular cholesterol leads to an upregulation of LDL-receptors and an increase in hepatic uptake of LDL-cholesterol from the circulation . These changes contribute to a decrease in circulating LDL-cholesterol levels, which is beneficial for patients with hypercholesterolemia .
Action Environment
The action of this compound can be influenced by various environmental factors. Additionally, the drug’s bioavailability and general circulation can be affected by first-pass metabolism in the liver and clearance by the digestive system . Understanding these factors can help optimize the drug’s efficacy and stability.
Biochemical Analysis
Biochemical Properties
Cerivastatin sodium interacts with the enzyme hydroxymethylglutaryl-coenzyme A (HMG-CoA) reductase, which is responsible for converting HMG-CoA to mevalonate . This interaction inhibits the enzyme, thereby reducing the endogenous synthesis of cholesterol .
Cellular Effects
This compound has a high affinity for liver tissue, the target site of action . By inhibiting HMG-CoA reductase in liver cells, this compound reduces the production of cholesterol, impacting cellular metabolism and potentially influencing cell signaling pathways and gene expression related to cholesterol synthesis and regulation .
Molecular Mechanism
The mechanism of action of this compound involves competitive inhibition of HMG-CoA reductase . By binding to the active site of this enzyme, this compound prevents the conversion of HMG-CoA to mevalonate, a crucial step in the biosynthesis of cholesterol .
Temporal Effects in Laboratory Settings
This compound is readily and completely absorbed from the gastrointestinal tract, with plasma concentrations reaching a peak 2 to 3 hours postadministration followed by a monoexponential decay with an elimination half-life (t½,β) of 2 to 3 hours . No accumulation is observed on repeated administration .
Dosage Effects in Animal Models
While specific dosage effects in animal models are not mentioned in the available literature, the pharmacokinetics of this compound are linear, with maximum plasma concentration (C max) and area under the concentration-time curve (AUC) proportional to the dose over the range of 0.05 to 0.8mg .
Metabolic Pathways
This compound is exclusively cleared via metabolism. No unchanged drug is excreted . It is subject to two main oxidative biotransformation reactions: demethylation of the benzylic methyl ether moiety leading to the metabolite M-1 and stereoselective hydroxylation of one methyl group of the 6-isopropyl substituent leading to the metabolite M-23 .
Transport and Distribution
This compound is highly bound to plasma proteins (>99%). The volume of distribution at steady state of about 0.3 L/kg indicates that the drug penetrates only moderately into tissue . Preclinical studies have shown a high affinity for liver tissue .
Preparation Methods
- Cerivastatin is synthesized through specific routes, but detailed synthetic procedures are not widely available.
- Industrial production methods involve chemical synthesis, purification, and formulation into dosage forms.
Chemical Reactions Analysis
- Cerivastatin undergoes various reactions, including oxidation, reduction, and substitution.
- Common reagents include oxidizing agents (e.g., KMnO₄), reducing agents (e.g., LiAlH₄), and nucleophilic substitution reagents (e.g., NaOH).
- Major products include cerivastatin itself and its metabolites.
Scientific Research Applications
Chemistry: Cerivastatin serves as a valuable tool for studying lipid metabolism and enzyme inhibition.
Biology: Researchers explore its effects on cell proliferation, apoptosis, and signaling pathways.
Medicine: Despite its withdrawal, cerivastatin’s impact on cardiovascular health remains a topic of interest.
Industry: Its synthesis and formulation contribute to drug development.
Comparison with Similar Compounds
- Cerivastatin’s uniqueness lies in its potency and tolerability.
- Similar compounds include other statins like atorvastatin, simvastatin, and rosuvastatin.
Biological Activity
Cerivastatin sodium, a synthetic statin, is primarily recognized for its potent ability to inhibit HMG-CoA reductase, an enzyme critical in cholesterol biosynthesis. This article delves into its biological activity, pharmacokinetics, and clinical implications, supported by data tables and relevant research findings.
Cerivastatin functions as a competitive inhibitor of HMG-CoA reductase. By blocking this enzyme, it effectively reduces the conversion of HMG-CoA to mevalonate, a precursor in the cholesterol synthesis pathway. This inhibition leads to decreased cholesterol levels in hepatic cells, which subsequently stimulates the synthesis of LDL receptors, enhancing the uptake of LDL particles from the bloodstream.
Key Mechanism Insights:
- Ki Value : Cerivastatin has a Ki value of , making it significantly more potent than lovastatin, which has a Ki value of .
- Inhibition in HepG2 Cells : The IC50 value for cerivastatin in human hepatoma cells is .
Pharmacokinetics
Cerivastatin is characterized by its rapid absorption and elimination profiles:
- Absorption : It is completely absorbed from the gastrointestinal tract with an absolute bioavailability of approximately 60% due to first-pass metabolism .
- Peak Plasma Concentration : Achieved 2 to 3 hours post-administration.
- Half-Life : The elimination half-life is about 2 to 3 hours .
- Protein Binding : Over 99% bound to plasma proteins, indicating limited tissue distribution outside the liver .
Table 1: Pharmacokinetic Parameters of Cerivastatin
Parameter | Value |
---|---|
Bioavailability | ~60% |
Peak Plasma Time | 2-3 hours |
Elimination Half-Life | 2-3 hours |
Protein Binding | >99% |
Volume of Distribution | ~0.3 L/kg |
Metabolism
Cerivastatin undergoes extensive hepatic metabolism primarily through cytochrome P450 enzymes (CYP2C8 and CYP3A4), producing active metabolites M-1 and M-23:
- Metabolite M-1 : Formed via demethylation.
- Metabolite M-23 : Formed via hydroxylation.
Both metabolites exhibit similar potency to cerivastatin itself .
Table 2: Metabolite Profiles
Metabolite | Formation Pathway | Activity Level |
---|---|---|
M-1 | Demethylation (CYP2C8/CYP3A4) | Active |
M-23 | Hydroxylation (CYP2C8) | Active |
M-24 | Combination of M-1 and M-23 | Not detectable in plasma |
Clinical Efficacy
Cerivastatin has been shown to significantly lower various lipid parameters:
- Total Cholesterol : Reduces plasma total cholesterol levels.
- LDL-C Reduction : Demonstrated reductions in low-density lipoprotein cholesterol (LDL-C) and apolipoprotein B.
- Triglycerides : Notable decreases in triglyceride levels were observed .
Case Studies
- Hypercholesterolemia Treatment :
- Atherosclerosis Progression :
Safety Profile and Side Effects
Despite its efficacy, cerivastatin was withdrawn from the market due to severe side effects including rhabdomyolysis when used in combination with certain other drugs. Monitoring for drug interactions remains critical due to its metabolism via CYP enzymes.
Properties
CAS No. |
143201-11-0 |
---|---|
Molecular Formula |
C26H34FNNaO5 |
Molecular Weight |
482.5 g/mol |
IUPAC Name |
sodium;(E,3R,5S)-7-[4-(4-fluorophenyl)-5-(methoxymethyl)-2,6-di(propan-2-yl)pyridin-3-yl]-3,5-dihydroxyhept-6-enoate |
InChI |
InChI=1S/C26H34FNO5.Na/c1-15(2)25-21(11-10-19(29)12-20(30)13-23(31)32)24(17-6-8-18(27)9-7-17)22(14-33-5)26(28-25)16(3)4;/h6-11,15-16,19-20,29-30H,12-14H2,1-5H3,(H,31,32);/b11-10+;/t19-,20-;/m1./s1 |
InChI Key |
UVXVKMCFVDNBCD-QCVDVZFFSA-N |
SMILES |
CC(C)C1=C(C(=C(C(=N1)C(C)C)COC)C2=CC=C(C=C2)F)C=CC(CC(CC(=O)[O-])O)O.[Na+] |
Isomeric SMILES |
CC(C)C1=C(C(=C(C(=N1)C(C)C)COC)C2=CC=C(C=C2)F)/C=C/[C@H](C[C@H](CC(=O)O)O)O.[Na] |
Canonical SMILES |
CC(C)C1=C(C(=C(C(=N1)C(C)C)COC)C2=CC=C(C=C2)F)C=CC(CC(CC(=O)O)O)O.[Na] |
Appearance |
Off-White Solid |
melting_point |
197-199°C |
Key on ui other cas no. |
143201-11-0 |
Purity |
> 95% |
quantity |
Milligrams-Grams |
Synonyms |
6-Heptenoic acid, 7-(4-(4-fluorophenyl)-5-(methoxymethyl)-2,6-bis(1-methylethyl)-3-pyridinyl)-3,5-dihydroxy-, monosodium salt, (S-(R*,S*-(E)))- 7-(4-(4-fluorophenyl)-2,6-diisopropyl-5-(methoxymethyl)pyrid-3-yl)-3,5-dihydroxy-6-heptenoate sodium salt Bay w 6228 Baycol cerivastatin cerivastatin sodium Certa Kazak Lipobay rivastatin |
Origin of Product |
United States |
Retrosynthesis Analysis
AI-Powered Synthesis Planning: Our tool employs the Template_relevance Pistachio, Template_relevance Bkms_metabolic, Template_relevance Pistachio_ringbreaker, Template_relevance Reaxys, Template_relevance Reaxys_biocatalysis model, leveraging a vast database of chemical reactions to predict feasible synthetic routes.
One-Step Synthesis Focus: Specifically designed for one-step synthesis, it provides concise and direct routes for your target compounds, streamlining the synthesis process.
Accurate Predictions: Utilizing the extensive PISTACHIO, BKMS_METABOLIC, PISTACHIO_RINGBREAKER, REAXYS, REAXYS_BIOCATALYSIS database, our tool offers high-accuracy predictions, reflecting the latest in chemical research and data.
Strategy Settings
Precursor scoring | Relevance Heuristic |
---|---|
Min. plausibility | 0.01 |
Model | Template_relevance |
Template Set | Pistachio/Bkms_metabolic/Pistachio_ringbreaker/Reaxys/Reaxys_biocatalysis |
Top-N result to add to graph | 6 |
Feasible Synthetic Routes
Q1: How does cerivastatin sodium exert its lipid-lowering effect?
A1: this compound is a synthetic, enantiomerically pure, 3-hydroxy-3-methylglutaryl-coenzyme A (HMG-CoA) reductase inhibitor. [, , ] HMG-CoA reductase is the rate-limiting enzyme in the mevalonate pathway, responsible for cholesterol biosynthesis. By inhibiting this enzyme, this compound effectively reduces cholesterol synthesis in the liver. This, in turn, leads to increased hepatic uptake of low-density lipoprotein cholesterol (LDL-C) from the bloodstream, ultimately lowering plasma cholesterol levels. [, , , ]
Q2: What is the molecular formula and weight of this compound?
A2: Unfortunately, the provided research papers do not specify the exact molecular formula and weight of this compound.
Q3: How is this compound metabolized, and does it interact with other drugs?
A3: this compound is primarily metabolized by the cytochrome P450 (CYP) enzyme, specifically CYP2C8, with a minor contribution from CYP3A4. [, ] It exhibits a dual metabolic pathway involving demethylation (metabolite M-1) and hydroxylation (metabolite M-23). [] Despite being a CYP3A4 substrate, this compound demonstrates a low potential for clinically relevant interactions with CYP3A4 inhibitors, such as erythromycin and itraconazole. [, ]
Q4: Does this compound interact with the immunosuppressant tacrolimus?
A4: Research indicates a moderate elevation of this compound systemic exposure when co-administered with tacrolimus in liver transplant recipients. [] While this interaction does not appear to be CYP3A4-mediated, the exact mechanism is thought to involve inhibition of active hepatic transport processes, particularly biliary excretion. []
Q5: What is the clinical efficacy of this compound in treating hypercholesterolemia?
A6: Clinical trials have demonstrated the effectiveness of this compound in lowering total cholesterol, LDL-C, and triglyceride levels in patients with primary hypercholesterolemia. [, , , ] A dose-dependent reduction in serum lipid levels was observed, with a higher dosage (0.3 mg/day) proving more effective than a lower dosage (0.15 mg/day) in patients with severe hypercholesterolemia. []
Q6: What are the safety concerns associated with this compound?
A7: While effective in lowering cholesterol levels, this compound has been associated with an increased risk of rhabdomyolysis, a serious condition involving muscle breakdown. [, ] This risk appears higher compared to other statins and is particularly pronounced when this compound is used in combination with gemfibrozil or at higher doses. [, ] These safety concerns ultimately led to the withdrawal of this compound from the market in 2001. [, ]
Q7: What analytical methods are used to quantify this compound in pharmaceutical formulations?
A8: High-performance liquid chromatography (HPLC) coupled with fluorescence detection is a commonly employed method for quantifying this compound and its metabolites in pharmaceutical preparations and biological samples. [, , ] This method offers high sensitivity and selectivity, allowing for accurate determination of drug concentrations. [, ]
Q8: Are there any known alternative compounds or approaches for achieving similar therapeutic effects?
A10: Yes, other HMG-CoA reductase inhibitors, collectively known as statins, are available and widely used for managing hypercholesterolemia. These include atorvastatin, simvastatin, pravastatin, and rosuvastatin. [, , , ] Each statin possesses a unique pharmacological profile, including potency, efficacy, and potential for adverse effects. [, , , ]
Q9: Has this compound shown any potential in other areas of research, such as cancer therapy?
A11: Interestingly, recent research suggests that this compound may act as a selective inhibitor of RNA polymerase I, an enzyme crucial for ribosome biogenesis and cell growth. [, ] Preliminary studies indicate antiproliferative effects of this compound against certain human cancer cell lines, hinting at its potential as a targeted anticancer therapeutic. [, ]
Disclaimer and Information on In-Vitro Research Products
Please be aware that all articles and product information presented on BenchChem are intended solely for informational purposes. The products available for purchase on BenchChem are specifically designed for in-vitro studies, which are conducted outside of living organisms. In-vitro studies, derived from the Latin term "in glass," involve experiments performed in controlled laboratory settings using cells or tissues. It is important to note that these products are not categorized as medicines or drugs, and they have not received approval from the FDA for the prevention, treatment, or cure of any medical condition, ailment, or disease. We must emphasize that any form of bodily introduction of these products into humans or animals is strictly prohibited by law. It is essential to adhere to these guidelines to ensure compliance with legal and ethical standards in research and experimentation.