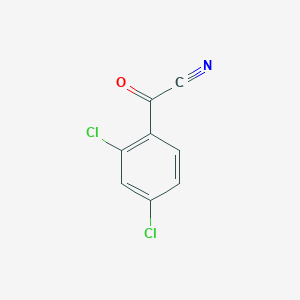
2,4-Dichlorobenzoyl Cyanide
Overview
Description
2,4-Dichlorobenzoyl Cyanide is a chemical compound that can be used in the preparation of benzoylcyclohexanedione pesticides . It is also an impurity in the synthesis of Lamotrigine .
Synthesis Analysis
The synthesis of 2,4-Dichlorobenzoyl Cyanide involves several steps. It is the key starting material for the synthesis of lamotrigine . The 2,3-dichlorobenzoyl cyanide has five regio isomers .Molecular Structure Analysis
The molecular formula of 2,4-Dichlorobenzoyl Cyanide is C8H3Cl2NO . Its average mass is 200.021 Da and its monoisotopic mass is 198.959167 Da .Scientific Research Applications
Silicone Rubber Manufacturing
Di (2,4-dichlorobenzoyl) peroxide (DCBP), a compound related to 2,4-Dichlorobenzoyl Cyanide, is commonly used in silicone rubber manufacturing as a crosslinking agent, vulcanizing agent, and polymerization initiator . It is advantageous because of its fast curing rate and bubble-free-cured transparent nature .
Thermal Decomposition Study
The thermal decomposition behavior of DCBP has been examined by combining simulations with thermal analysis methods . This helps in understanding the foundation of thermokinetics, such as the peak temperature, heat of decomposition, and apparent activation energy of DCBP .
Thermal Ignition Theory Application
The application of thermal ignition theory of DCBP has been studied by kinetic-based curve fitting . This represents a major advancement in the comprehension of behavior between heat release and heat transfer to the surroundings incorporated into a single differential equation .
Safety Analysis
Due to its reactivity or incompatibility, DCBP may negatively affect safety requirements and concerns during chemical reactions . Therefore, its properties have been investigated using differential scanning calorimetry and a literature review .
Decomposition Characteristics Study
The decomposition characteristics of DCBP have been studied using isothermal and non-isothermal calorimetric techniques combined with a simulation approach . This helps in predicting unwarranted runaway reactions, which entail enormous pressure rise and release of toxic by-products to the environment .
Production of Silicone Products
Despite DCBP decomposition deposits with dioxins, which require major decontamination measures, DCBP is used to produce silicone products globally . The present study establishes threshold thermokinetic parameters for packing and handling thermally sensitive organic peroxides .
Mechanism of Action
Target of Action
2,4-Dichlorobenzoyl Cyanide (DCBC) is an organic compound that is primarily used as an intermediate in the production of dyes and pharmaceuticals It’s known that dcbc interacts with various biochemical processes during its use in the production of dyes and pharmaceuticals .
Mode of Action
It’s known that dcbc undergoes thermal decomposition, which is an autocatalytic reaction . This means that the reaction accelerates as it proceeds, leading to a rapid release of energy . This property is crucial in its role as an initiator, hardener, and vulcanizing agent in the rubber industry .
Biochemical Pathways
This process can lead to various downstream effects, including the release of energy and the formation of new compounds .
Result of Action
The primary result of DCBC’s action is its role in the production of dyes and pharmaceuticals . It’s also used as an initiator, hardener, and vulcanizing agent in the rubber industry . The thermal decomposition of DCBC leads to a rapid release of energy, which is crucial in these applications .
Action Environment
The action of DCBC can be influenced by various environmental factors. For instance, the thermal decomposition of DCBC is a temperature-dependent process . Therefore, the rate and extent of this reaction can be influenced by the temperature of the environment . Additionally, safety precautions must be taken to prevent the release of DCBC into the environment, as it can pose potential hazards .
Safety and Hazards
2,4-Dichlorobenzoyl Cyanide is considered hazardous. It causes severe skin burns and eye damage . It reacts violently with water and contact with water liberates toxic gas . Safety measures include not breathing dust/fume/gas/mist/vapors/spray, washing face, hands and any exposed skin thoroughly after handling, wearing protective gloves/clothing/eye protection/face protection, and storing locked up .
properties
IUPAC Name |
2,4-dichlorobenzoyl cyanide | |
---|---|---|
Source | PubChem | |
URL | https://pubchem.ncbi.nlm.nih.gov | |
Description | Data deposited in or computed by PubChem | |
InChI |
InChI=1S/C8H3Cl2NO/c9-5-1-2-6(7(10)3-5)8(12)4-11/h1-3H | |
Source | PubChem | |
URL | https://pubchem.ncbi.nlm.nih.gov | |
Description | Data deposited in or computed by PubChem | |
InChI Key |
MJJCNUBDKYFMHM-UHFFFAOYSA-N | |
Source | PubChem | |
URL | https://pubchem.ncbi.nlm.nih.gov | |
Description | Data deposited in or computed by PubChem | |
Canonical SMILES |
C1=CC(=C(C=C1Cl)Cl)C(=O)C#N | |
Source | PubChem | |
URL | https://pubchem.ncbi.nlm.nih.gov | |
Description | Data deposited in or computed by PubChem | |
Molecular Formula |
C8H3Cl2NO | |
Source | PubChem | |
URL | https://pubchem.ncbi.nlm.nih.gov | |
Description | Data deposited in or computed by PubChem | |
DSSTOX Substance ID |
DTXSID20431458 | |
Record name | 2,4-Dichlorobenzoyl Cyanide | |
Source | EPA DSSTox | |
URL | https://comptox.epa.gov/dashboard/DTXSID20431458 | |
Description | DSSTox provides a high quality public chemistry resource for supporting improved predictive toxicology. | |
Molecular Weight |
200.02 g/mol | |
Source | PubChem | |
URL | https://pubchem.ncbi.nlm.nih.gov | |
Description | Data deposited in or computed by PubChem | |
CAS RN |
35022-43-6 | |
Record name | 2,4-Dichlorobenzoyl cyanide | |
Source | CAS Common Chemistry | |
URL | https://commonchemistry.cas.org/detail?cas_rn=35022-43-6 | |
Description | CAS Common Chemistry is an open community resource for accessing chemical information. Nearly 500,000 chemical substances from CAS REGISTRY cover areas of community interest, including common and frequently regulated chemicals, and those relevant to high school and undergraduate chemistry classes. This chemical information, curated by our expert scientists, is provided in alignment with our mission as a division of the American Chemical Society. | |
Explanation | The data from CAS Common Chemistry is provided under a CC-BY-NC 4.0 license, unless otherwise stated. | |
Record name | 2,4-Dichlorobenzoyl Cyanide | |
Source | EPA DSSTox | |
URL | https://comptox.epa.gov/dashboard/DTXSID20431458 | |
Description | DSSTox provides a high quality public chemistry resource for supporting improved predictive toxicology. | |
Synthesis routes and methods I
Procedure details
Synthesis routes and methods II
Procedure details
Retrosynthesis Analysis
AI-Powered Synthesis Planning: Our tool employs the Template_relevance Pistachio, Template_relevance Bkms_metabolic, Template_relevance Pistachio_ringbreaker, Template_relevance Reaxys, Template_relevance Reaxys_biocatalysis model, leveraging a vast database of chemical reactions to predict feasible synthetic routes.
One-Step Synthesis Focus: Specifically designed for one-step synthesis, it provides concise and direct routes for your target compounds, streamlining the synthesis process.
Accurate Predictions: Utilizing the extensive PISTACHIO, BKMS_METABOLIC, PISTACHIO_RINGBREAKER, REAXYS, REAXYS_BIOCATALYSIS database, our tool offers high-accuracy predictions, reflecting the latest in chemical research and data.
Strategy Settings
Precursor scoring | Relevance Heuristic |
---|---|
Min. plausibility | 0.01 |
Model | Template_relevance |
Template Set | Pistachio/Bkms_metabolic/Pistachio_ringbreaker/Reaxys/Reaxys_biocatalysis |
Top-N result to add to graph | 6 |
Feasible Synthetic Routes
Disclaimer and Information on In-Vitro Research Products
Please be aware that all articles and product information presented on BenchChem are intended solely for informational purposes. The products available for purchase on BenchChem are specifically designed for in-vitro studies, which are conducted outside of living organisms. In-vitro studies, derived from the Latin term "in glass," involve experiments performed in controlled laboratory settings using cells or tissues. It is important to note that these products are not categorized as medicines or drugs, and they have not received approval from the FDA for the prevention, treatment, or cure of any medical condition, ailment, or disease. We must emphasize that any form of bodily introduction of these products into humans or animals is strictly prohibited by law. It is essential to adhere to these guidelines to ensure compliance with legal and ethical standards in research and experimentation.