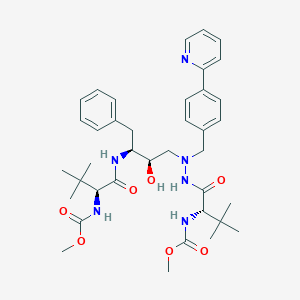
8-epi Atazanavir
Overview
Description
8-epi Atazanavir is a stereoisomer of Atazanavir, a well-known antiretroviral medication used in the treatment of HIV/AIDS. Atazanavir belongs to the class of protease inhibitors, which function by inhibiting the protease enzyme, thereby preventing the maturation of viral particles. The “8-epi” designation indicates a specific stereochemical configuration at the eighth position of the molecule, which can influence its biological activity and pharmacokinetic properties.
Mechanism of Action
Target of Action
8-epi Atazanavir, like Atazanavir, is an antiretroviral drug of the protease inhibitor (PI) class . It selectively inhibits the HIV-1 protease , an enzyme critical for HIV-1 virion maturation . This enzyme is responsible for the virus-specific processing of viral Gag and Gag-Pol polyproteins in HIV-1 infected cells .
Mode of Action
This compound binds to the active site of the HIV-1 protease . This binding inhibits the cleavage of viral Gag and Gag-Pol polyprotein precursors into individual functional proteins required for infectious HIV . As a result, the formation of mature virions is prevented .
Biochemical Pathways
The major biotransformation pathways of Atazanavir in humans consist of monooxygenation and dioxygenation . Other minor biotransformation pathways for Atazanavir or its metabolites consist of glucuronidation, N-dealkylation, hydrolysis, and oxygenation with dehydrogenation . Atazanavir is primarily and extensively metabolized by the cytochrome P450, family 3, subfamily A (CYP3A) polypeptide isoenzymes 4 and 5 (CYP3A4/5) .
Pharmacokinetics
Atazanavir is rapidly absorbed and peak concentration is reached between 2 – 2.5 hours post-dose . Its absorption is improved when it is taken with food . The primary route of Atazanavir elimination is through bile (79% of administered dose) and the secondary route is through urine (13% of administered dose excreted as metabolites) . Atazanavir demonstrates nonlinear pharmacokinetics with greater than dose-proportional increases in AUC and C max values over the dose range of 200 to 800 mg once daily .
Result of Action
The inhibition of the HIV-1 protease by this compound prevents the formation of mature virions, resulting in the production of immature, noninfectious viral particles . This effectively reduces the number of infectious particles in the body, slowing the progression of the disease.
Action Environment
The effectiveness of this compound can be influenced by various environmental factors. For instance, Atazanavir solubility decreases as pH increases . Reduced plasma concentrations of Atazanavir occur if antacids, buffered medications, H2-receptor antagonists, and proton-pump inhibitors are administered with Atazanavir . Therefore, it is recommended to avoid concomitant use . Furthermore, administration of Atazanavir with food enhances bioavailability (35-70% ↑ AUC) and reduces pharmacokinetic variability by 50% .
Biochemical Analysis
Biochemical Properties
8-epi Atazanavir plays a significant role in biochemical reactions by interacting with various enzymes, proteins, and other biomolecules. It primarily inhibits the HIV-1 protease enzyme, which is crucial for the viral replication process. By binding to the active site of the protease enzyme, this compound prevents the cleavage of viral Gag and Gag-Pol polyproteins, thereby inhibiting the formation of mature virions . Additionally, this compound has been shown to interact with cytochrome P450 enzymes, particularly CYP3A4, which is involved in its metabolism .
Cellular Effects
This compound exerts various effects on different types of cells and cellular processes. In HIV-infected cells, it inhibits viral replication by preventing the maturation of viral particles. This inhibition leads to a decrease in viral load and an improvement in immune function. This compound also influences cell signaling pathways, gene expression, and cellular metabolism. It has been observed to cause elevations in serum aminotransferase levels and indirect hyperbilirubinemia, indicating its impact on liver cells . Furthermore, this compound affects the expression of genes involved in drug metabolism and transport .
Molecular Mechanism
The molecular mechanism of this compound involves its binding interactions with biomolecules and enzyme inhibition. By binding to the active site of the HIV-1 protease enzyme, this compound inhibits the cleavage of viral polyproteins, preventing the formation of mature and infectious viral particles . This inhibition disrupts the viral life cycle and reduces viral replication. Additionally, this compound interacts with cytochrome P450 enzymes, leading to its metabolism and subsequent elimination from the body .
Temporal Effects in Laboratory Settings
In laboratory settings, the effects of this compound have been studied over time to understand its stability, degradation, and long-term effects on cellular function. It has been observed that this compound remains stable under controlled conditions, with minimal degradation over extended periods . Long-term studies have shown that this compound maintains its inhibitory effects on viral replication and continues to reduce viral load in HIV-infected cells . Prolonged exposure to this compound may lead to the development of drug resistance in some viral strains .
Dosage Effects in Animal Models
The effects of this compound at different dosages have been studied in animal models to determine its therapeutic window and potential toxic effects. In these studies, it has been observed that this compound exhibits dose-dependent antiviral activity, with higher doses leading to greater inhibition of viral replication . At high doses, this compound may cause toxic effects, including hepatotoxicity and gastrointestinal disturbances . These findings highlight the importance of optimizing the dosage to achieve maximum therapeutic efficacy while minimizing adverse effects.
Metabolic Pathways
This compound is involved in various metabolic pathways, primarily mediated by cytochrome P450 enzymes. The major biotransformation pathways of this compound include monooxygenation and dioxygenation . Additionally, minor pathways such as glucuronidation, N-dealkylation, hydrolysis, and oxygenation with dehydrogenation contribute to its metabolism . These metabolic processes result in the formation of several metabolites, which are subsequently eliminated from the body .
Transport and Distribution
The transport and distribution of this compound within cells and tissues are regulated by various transporters and binding proteins. P-glycoprotein and organic anion transporting polypeptides (OATPs) play a crucial role in the intestinal absorption and distribution of this compound . These transporters facilitate the uptake and efflux of this compound across cellular membranes, influencing its bioavailability and tissue distribution . Additionally, this compound exhibits high protein binding, primarily to alpha-1-acid glycoprotein and albumin .
Subcellular Localization
The subcellular localization of this compound is primarily within the cytoplasm of infected cells. It targets the HIV-1 protease enzyme, which is localized in the cytoplasm, to exert its inhibitory effects . Additionally, this compound may undergo post-translational modifications that direct it to specific cellular compartments or organelles . These modifications play a role in its activity and function within the cell.
Preparation Methods
Synthetic Routes and Reaction Conditions: The synthesis of 8-epi Atazanavir involves several key steps, including the formation of the core structure and the introduction of various functional groups. The process typically begins with the preparation of a chiral intermediate, which is then subjected to a series of reactions to build the final compound. Common reagents used in these steps include protecting groups, coupling agents, and chiral catalysts to ensure the correct stereochemistry is achieved.
Industrial Production Methods: Industrial production of this compound follows similar synthetic routes but on a larger scale. The process is optimized for yield, purity, and cost-effectiveness. Techniques such as high-performance liquid chromatography (HPLC) are employed to purify the final product, ensuring it meets the stringent standards required for pharmaceutical use.
Chemical Reactions Analysis
Types of Reactions: 8-epi Atazanavir undergoes various chemical reactions, including:
Oxidation: Introduction of oxygen atoms into the molecule, often using reagents like hydrogen peroxide or potassium permanganate.
Reduction: Removal of oxygen atoms or addition of hydrogen atoms, typically using reagents like lithium aluminum hydride or sodium borohydride.
Substitution: Replacement of one functional group with another, using reagents such as halides or nucleophiles.
Common Reagents and Conditions:
Oxidation: Hydrogen peroxide, potassium permanganate.
Reduction: Lithium aluminum hydride, sodium borohydride.
Substitution: Halides, nucleophiles.
Major Products Formed: The major products formed from these reactions depend on the specific conditions and reagents used. For example, oxidation may yield hydroxylated derivatives, while reduction could produce deoxygenated analogs.
Scientific Research Applications
8-epi Atazanavir has several scientific research applications, including:
Chemistry: Used as a model compound to study stereochemistry and reaction mechanisms.
Biology: Investigated for its potential effects on various biological pathways and its interaction with proteins.
Medicine: Explored for its efficacy in treating HIV/AIDS and other viral infections.
Industry: Utilized in the development of new pharmaceutical formulations and drug delivery systems.
Comparison with Similar Compounds
Atazanavir: The parent compound, widely used in HIV treatment.
Darunavir: Another protease inhibitor with a similar mechanism of action.
Lopinavir: Often used in combination with ritonavir for enhanced efficacy.
Comparison: 8-epi Atazanavir is unique due to its specific stereochemical configuration, which can affect its pharmacokinetic properties and biological activity. Compared to Atazanavir, this compound may exhibit different binding affinities and metabolic profiles, potentially leading to variations in efficacy and side effects. When compared to other protease inhibitors like Darunavir and Lopinavir, this compound offers an alternative option with distinct molecular characteristics that may be advantageous in certain therapeutic contexts.
Properties
IUPAC Name |
methyl N-[(2S)-1-[2-[(2R,3S)-2-hydroxy-3-[[(2S)-2-(methoxycarbonylamino)-3,3-dimethylbutanoyl]amino]-4-phenylbutyl]-2-[(4-pyridin-2-ylphenyl)methyl]hydrazinyl]-3,3-dimethyl-1-oxobutan-2-yl]carbamate | |
---|---|---|
Source | PubChem | |
URL | https://pubchem.ncbi.nlm.nih.gov | |
Description | Data deposited in or computed by PubChem | |
InChI |
InChI=1S/C38H52N6O7/c1-37(2,3)31(41-35(48)50-7)33(46)40-29(22-25-14-10-9-11-15-25)30(45)24-44(43-34(47)32(38(4,5)6)42-36(49)51-8)23-26-17-19-27(20-18-26)28-16-12-13-21-39-28/h9-21,29-32,45H,22-24H2,1-8H3,(H,40,46)(H,41,48)(H,42,49)(H,43,47)/t29-,30+,31+,32+/m0/s1 | |
Source | PubChem | |
URL | https://pubchem.ncbi.nlm.nih.gov | |
Description | Data deposited in or computed by PubChem | |
InChI Key |
AXRYRYVKAWYZBR-SYEZAVJTSA-N | |
Source | PubChem | |
URL | https://pubchem.ncbi.nlm.nih.gov | |
Description | Data deposited in or computed by PubChem | |
Canonical SMILES |
CC(C)(C)C(C(=O)NC(CC1=CC=CC=C1)C(CN(CC2=CC=C(C=C2)C3=CC=CC=N3)NC(=O)C(C(C)(C)C)NC(=O)OC)O)NC(=O)OC | |
Source | PubChem | |
URL | https://pubchem.ncbi.nlm.nih.gov | |
Description | Data deposited in or computed by PubChem | |
Isomeric SMILES |
CC(C)(C)[C@@H](C(=O)N[C@@H](CC1=CC=CC=C1)[C@@H](CN(CC2=CC=C(C=C2)C3=CC=CC=N3)NC(=O)[C@H](C(C)(C)C)NC(=O)OC)O)NC(=O)OC | |
Source | PubChem | |
URL | https://pubchem.ncbi.nlm.nih.gov | |
Description | Data deposited in or computed by PubChem | |
Molecular Formula |
C38H52N6O7 | |
Source | PubChem | |
URL | https://pubchem.ncbi.nlm.nih.gov | |
Description | Data deposited in or computed by PubChem | |
DSSTOX Substance ID |
DTXSID40629138 | |
Record name | Methyl [(5S,10R,11S,14S)-11-benzyl-5-tert-butyl-10-hydroxy-15,15-dimethyl-3,6,13-trioxo-8-{[4-(pyridin-2-yl)phenyl]methyl}-2-oxa-4,7,8,12-tetraazahexadecan-14-yl]carbamate (non-preferred name) | |
Source | EPA DSSTox | |
URL | https://comptox.epa.gov/dashboard/DTXSID40629138 | |
Description | DSSTox provides a high quality public chemistry resource for supporting improved predictive toxicology. | |
Molecular Weight |
704.9 g/mol | |
Source | PubChem | |
URL | https://pubchem.ncbi.nlm.nih.gov | |
Description | Data deposited in or computed by PubChem | |
CAS No. |
1292296-09-3 | |
Record name | Atazanavir S,R,S,S-diastereomer | |
Source | ChemIDplus | |
URL | https://pubchem.ncbi.nlm.nih.gov/substance/?source=chemidplus&sourceid=1292296093 | |
Description | ChemIDplus is a free, web search system that provides access to the structure and nomenclature authority files used for the identification of chemical substances cited in National Library of Medicine (NLM) databases, including the TOXNET system. | |
Record name | Methyl [(5S,10R,11S,14S)-11-benzyl-5-tert-butyl-10-hydroxy-15,15-dimethyl-3,6,13-trioxo-8-{[4-(pyridin-2-yl)phenyl]methyl}-2-oxa-4,7,8,12-tetraazahexadecan-14-yl]carbamate (non-preferred name) | |
Source | EPA DSSTox | |
URL | https://comptox.epa.gov/dashboard/DTXSID40629138 | |
Description | DSSTox provides a high quality public chemistry resource for supporting improved predictive toxicology. | |
Record name | ATAZANAVIR S,R,S,S-DIASTEREOMER | |
Source | FDA Global Substance Registration System (GSRS) | |
URL | https://gsrs.ncats.nih.gov/ginas/app/beta/substances/5EQX656EUV | |
Description | The FDA Global Substance Registration System (GSRS) enables the efficient and accurate exchange of information on what substances are in regulated products. Instead of relying on names, which vary across regulatory domains, countries, and regions, the GSRS knowledge base makes it possible for substances to be defined by standardized, scientific descriptions. | |
Explanation | Unless otherwise noted, the contents of the FDA website (www.fda.gov), both text and graphics, are not copyrighted. They are in the public domain and may be republished, reprinted and otherwise used freely by anyone without the need to obtain permission from FDA. Credit to the U.S. Food and Drug Administration as the source is appreciated but not required. | |
Retrosynthesis Analysis
AI-Powered Synthesis Planning: Our tool employs the Template_relevance Pistachio, Template_relevance Bkms_metabolic, Template_relevance Pistachio_ringbreaker, Template_relevance Reaxys, Template_relevance Reaxys_biocatalysis model, leveraging a vast database of chemical reactions to predict feasible synthetic routes.
One-Step Synthesis Focus: Specifically designed for one-step synthesis, it provides concise and direct routes for your target compounds, streamlining the synthesis process.
Accurate Predictions: Utilizing the extensive PISTACHIO, BKMS_METABOLIC, PISTACHIO_RINGBREAKER, REAXYS, REAXYS_BIOCATALYSIS database, our tool offers high-accuracy predictions, reflecting the latest in chemical research and data.
Strategy Settings
Precursor scoring | Relevance Heuristic |
---|---|
Min. plausibility | 0.01 |
Model | Template_relevance |
Template Set | Pistachio/Bkms_metabolic/Pistachio_ringbreaker/Reaxys/Reaxys_biocatalysis |
Top-N result to add to graph | 6 |
Feasible Synthetic Routes
Disclaimer and Information on In-Vitro Research Products
Please be aware that all articles and product information presented on BenchChem are intended solely for informational purposes. The products available for purchase on BenchChem are specifically designed for in-vitro studies, which are conducted outside of living organisms. In-vitro studies, derived from the Latin term "in glass," involve experiments performed in controlled laboratory settings using cells or tissues. It is important to note that these products are not categorized as medicines or drugs, and they have not received approval from the FDA for the prevention, treatment, or cure of any medical condition, ailment, or disease. We must emphasize that any form of bodily introduction of these products into humans or animals is strictly prohibited by law. It is essential to adhere to these guidelines to ensure compliance with legal and ethical standards in research and experimentation.