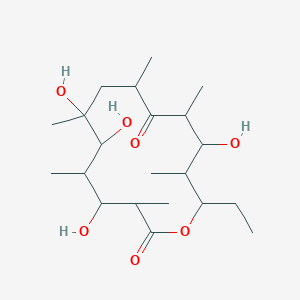
Erythronolide B
Overview
Description
Erythronolide B is a macrolide compound that serves as a crucial intermediate in the biosynthesis of erythromycin, a widely used antibiotic. It is a 14-membered lactone ring structure with multiple chiral centers, making it a complex molecule of significant interest in organic chemistry and pharmaceutical research .
Mechanism of Action
Target of Action
The primary target of Erythronolide B is the 6-deoxythis compound hydroxylase . This enzyme is found in the organism Saccharopolyspora erythraea , which is a soil-dwelling actinomycete .
Mode of Action
This compound interacts with its target, the 6-deoxythis compound hydroxylase, through a NADPH-dependent conversion . This interaction involves the insertion of an oxygen atom at the 6S position of 6-deoxythis compound . This process requires the participation of a ferredoxin .
Biochemical Pathways
The biosynthesis of this compound involves a series of enzymatic reactions. It starts with the reaction of propionyl CoA with methylmalonyl CoA, generating a compound enantioselectively . This compound undergoes diastereoselective reduction to deliver a β-hydroxy thioester . The resulting β-ketothioester is reduced to a β-hydroxyester, which reacts with additional methylmalonyl CoA and NADPH to generate a precursor . Lactonization then provides 6-deoxythis compound, from which this compound is formed by oxidation .
Result of Action
The result of this compound’s action is the formation of this compound (EB) from 6-deoxythis compound (6-DEB) through the insertion of an oxygen atom at the 6S position of 6-DEB . This process results in a molecule with significant biological activity.
Action Environment
The action of this compound is influenced by environmental factors. For instance, the enzyme 6-deoxythis compound hydroxylase, which is the target of this compound, is found in the soil-dwelling actinomycete Saccharopolyspora erythraea . This suggests that the action of this compound may be influenced by the conditions in the soil environment where this organism is found.
Biochemical Analysis
Biochemical Properties
Erythronolide B plays a significant role in biochemical reactions, particularly in the biosynthesis of erythromycin . It is synthesized from acyl-CoA precursors by polyketide synthases (PKSs), a process that involves a series of enzymatic reactions . The enzymes involved in this process include acyl transferase (AT), acyl-carrier protein (ACP), and ketosynthase (KS) domains . The cytochrome P450 oxidase EryF catalyzes the C6 hydroxylation of 6-deoxythis compound to form this compound .
Cellular Effects
In Escherichia coli, the production of the polyketide skeleton 6-deoxythis compound, a precursor to this compound, has been demonstrated . The production of this compound influences the cellular metabolism of E. coli, leading to changes in cell function
Molecular Mechanism
The molecular mechanism of this compound involves a series of enzymatic reactions. The cytochrome P450 oxidase EryF plays a crucial role in the formation of this compound. It catalyzes the C6 hydroxylation of 6-deoxythis compound, which is the initial rate-limiting modification in a multi-step pathway to convert 6-deoxythis compound into erythromycin .
Metabolic Pathways
This compound is involved in the metabolic pathway of erythromycin biosynthesis . The biosynthetic pathways of L-mycarose, D-desosamine, and methylmalonyl-CoA and methylpropionyl-CoA precursors of this compound have been reviewed .
Preparation Methods
Synthetic Routes and Reaction Conditions: The synthesis of erythronolide B typically involves the enzyme-catalyzed reaction of propionyl coenzyme A with methylmalonyl coenzyme A. This reaction generates a series of intermediates through diastereoselective reductions and condensations, ultimately forming the macrolide structure . The process includes the following steps:
Enzyme-catalyzed reactions: Propionyl coenzyme A reacts with methylmalonyl coenzyme A to form a β-hydroxy thioester.
Reduction and condensation: The β-hydroxy thioester undergoes reduction and Claisen condensation to form the seco acid precursor.
Lactonization: The seco acid precursor undergoes lactonization to form 6-deoxythis compound, which is then oxidized to this compound.
Industrial Production Methods: Industrial production of this compound often involves fermentation processes using genetically engineered strains of Saccharopolyspora erythraea. These strains are optimized to produce high yields of this compound through the manipulation of metabolic pathways and enzyme activities .
Chemical Reactions Analysis
Types of Reactions: Erythronolide B undergoes various chemical reactions, including:
Oxidation: Conversion of 6-deoxythis compound to this compound through the insertion of an oxygen atom.
Reduction: Diastereoselective reduction of intermediates during its biosynthesis.
Substitution: Functional group modifications to form derivatives such as erythromycin.
Common Reagents and Conditions:
Oxidizing agents: Used in the conversion of 6-deoxythis compound to this compound.
Reducing agents: Employed in the diastereoselective reduction steps.
Enzymes: Catalyze various steps in the biosynthesis pathway.
Major Products:
Erythromycin: A broad-spectrum antibiotic derived from this compound.
6-Deoxythis compound: An intermediate in the biosynthesis of this compound.
Scientific Research Applications
Erythronolide B has numerous applications in scientific research:
Chemistry: Used as a model compound for studying complex macrolide synthesis and enzyme-catalyzed reactions.
Biology: Investigated for its role in the biosynthesis of erythromycin and other macrolide antibiotics.
Medicine: Serves as a precursor for erythromycin, which is used to treat various bacterial infections.
Industry: Employed in the production of erythromycin and its derivatives through fermentation and chemical synthesis
Comparison with Similar Compounds
6-Deoxyerythronolide B: A direct precursor in the biosynthesis of this compound.
Erythronolide A: Another macrolide compound with a similar structure but different functional groups.
9(S)-Dihydroerythronolide A: A derivative with modifications at specific positions on the lactone ring.
Uniqueness: this compound is unique due to its specific role as an intermediate in the biosynthesis of erythromycin. Its complex structure with multiple chiral centers makes it a challenging and valuable target for synthetic organic chemistry .
Properties
IUPAC Name |
14-ethyl-4,6,7,12-tetrahydroxy-3,5,7,9,11,13-hexamethyl-oxacyclotetradecane-2,10-dione | |
---|---|---|
Details | Computed by LexiChem 2.6.6 (PubChem release 2019.06.18) | |
Source | PubChem | |
URL | https://pubchem.ncbi.nlm.nih.gov | |
Description | Data deposited in or computed by PubChem | |
InChI |
InChI=1S/C21H38O7/c1-8-15-11(3)17(23)12(4)16(22)10(2)9-21(7,27)19(25)13(5)18(24)14(6)20(26)28-15/h10-15,17-19,23-25,27H,8-9H2,1-7H3 | |
Details | Computed by InChI 1.0.5 (PubChem release 2019.06.18) | |
Source | PubChem | |
URL | https://pubchem.ncbi.nlm.nih.gov | |
Description | Data deposited in or computed by PubChem | |
InChI Key |
ZFBRGCCVTUPRFQ-UHFFFAOYSA-N | |
Details | Computed by InChI 1.0.5 (PubChem release 2019.06.18) | |
Source | PubChem | |
URL | https://pubchem.ncbi.nlm.nih.gov | |
Description | Data deposited in or computed by PubChem | |
Canonical SMILES |
CCC1C(C(C(C(=O)C(CC(C(C(C(C(C(=O)O1)C)O)C)O)(C)O)C)C)O)C | |
Details | Computed by OEChem 2.1.5 (PubChem release 2019.06.18) | |
Source | PubChem | |
URL | https://pubchem.ncbi.nlm.nih.gov | |
Description | Data deposited in or computed by PubChem | |
Molecular Formula |
C21H38O7 | |
Details | Computed by PubChem 2.1 (PubChem release 2019.06.18) | |
Source | PubChem | |
URL | https://pubchem.ncbi.nlm.nih.gov | |
Description | Data deposited in or computed by PubChem | |
DSSTOX Substance ID |
DTXSID20275990 | |
Record name | Erythronolide A, 12-deoxy- | |
Source | EPA DSSTox | |
URL | https://comptox.epa.gov/dashboard/DTXSID20275990 | |
Description | DSSTox provides a high quality public chemistry resource for supporting improved predictive toxicology. | |
Molecular Weight |
402.5 g/mol | |
Details | Computed by PubChem 2.1 (PubChem release 2021.05.07) | |
Source | PubChem | |
URL | https://pubchem.ncbi.nlm.nih.gov | |
Description | Data deposited in or computed by PubChem | |
CAS No. |
3225-82-9, 19270-26-9 | |
Record name | Erythronolide B | |
Source | ChemIDplus | |
URL | https://pubchem.ncbi.nlm.nih.gov/substance/?source=chemidplus&sourceid=0003225829 | |
Description | ChemIDplus is a free, web search system that provides access to the structure and nomenclature authority files used for the identification of chemical substances cited in National Library of Medicine (NLM) databases, including the TOXNET system. | |
Record name | Erythronolide A, 12-deoxy- | |
Source | EPA DSSTox | |
URL | https://comptox.epa.gov/dashboard/DTXSID20275990 | |
Description | DSSTox provides a high quality public chemistry resource for supporting improved predictive toxicology. | |
Retrosynthesis Analysis
AI-Powered Synthesis Planning: Our tool employs the Template_relevance Pistachio, Template_relevance Bkms_metabolic, Template_relevance Pistachio_ringbreaker, Template_relevance Reaxys, Template_relevance Reaxys_biocatalysis model, leveraging a vast database of chemical reactions to predict feasible synthetic routes.
One-Step Synthesis Focus: Specifically designed for one-step synthesis, it provides concise and direct routes for your target compounds, streamlining the synthesis process.
Accurate Predictions: Utilizing the extensive PISTACHIO, BKMS_METABOLIC, PISTACHIO_RINGBREAKER, REAXYS, REAXYS_BIOCATALYSIS database, our tool offers high-accuracy predictions, reflecting the latest in chemical research and data.
Strategy Settings
Precursor scoring | Relevance Heuristic |
---|---|
Min. plausibility | 0.01 |
Model | Template_relevance |
Template Set | Pistachio/Bkms_metabolic/Pistachio_ringbreaker/Reaxys/Reaxys_biocatalysis |
Top-N result to add to graph | 6 |
Feasible Synthetic Routes
Q1: What is the role of erythronolide B in erythromycin biosynthesis?
A1: this compound serves as the central aglycone core in erythromycin biosynthesis. It is further modified by the attachment of sugar moieties and enzymatic tailoring to yield the final active antibiotic, erythromycin A [, , , ].
Q2: How is this compound produced in nature?
A2: Saccharopolyspora erythraea, a bacterium, biosynthesizes this compound through a multi-step process utilizing a polyketide synthase (PKS) enzyme complex encoded by the ery gene cluster. This complex assembles propionyl-CoA and methylmalonyl-CoA building blocks to form the macrolactone ring [, , , ].
Q3: What is the molecular formula and weight of this compound?
A3: The molecular formula of this compound is C21H38O6, and its molecular weight is 386.52 g/mol.
Q4: Are there any spectroscopic techniques used to characterize this compound?
A4: Yes, various spectroscopic methods are employed to elucidate the structure and confirm the identity of this compound. These include:
- Nuclear Magnetic Resonance (NMR) Spectroscopy: Provides detailed information on the connectivity and spatial arrangement of atoms within the molecule, confirming stereochemistry [].
- Mass Spectrometry (MS): Determines the molecular weight and fragmentation pattern, aiding in structural elucidation and compound identification [, , ].
Q5: What are the key enzymes involved in modifying this compound during erythromycin biosynthesis?
A5: Several enzymes play crucial roles in modifying this compound:
- 6-Deoxythis compound Hydroxylase (EryF): Catalyzes the hydroxylation of 6-deoxythis compound to form this compound, a critical step in erythromycin A biosynthesis [, , ].
- Glycosyltransferases: These enzymes, including EryBV (mycarosyltransferase) and EryCIII (desosaminyltransferase), attach sugar moieties like L-mycarose and D-desosamine to specific positions on this compound, contributing to the final structure and activity of erythromycin A [, , ].
Q6: Can this compound be used to generate novel erythromycin derivatives?
A6: Yes, this compound serves as a valuable starting point for generating novel erythromycin derivatives through combinatorial biosynthesis. This approach involves engineering the biosynthetic pathway in Saccharopolyspora erythraea or utilizing heterologous expression systems to introduce alternative sugar moieties or modify existing ones on the this compound scaffold [, , , ].
Q7: What are some examples of novel compounds generated using this compound as a precursor?
A7: Researchers have successfully produced several novel compounds using this compound, including:
- Hybrid Macrolides: By introducing glycosyltransferases from other antibiotic-producing bacteria into Saccharopolyspora erythraea strains, researchers have generated hybrid macrolides with altered sugar profiles, potentially impacting their activity and stability [, ].
- Methylated Erythromycin Derivatives: Expression of sugar O-methyltransferases from the spinosyn biosynthetic pathway in Saccharopolyspora erythraea mutants has led to the production of erythromycin derivatives with methylated sugar residues, influencing their activity against specific bacterial strains [].
Q8: How does the structure of this compound influence the activity of the enzymes involved in its modification?
A8: The specific hydroxyl groups and their stereochemistry in the this compound structure are essential for recognition and catalysis by enzymes like EryF and glycosyltransferases. Modifications to these groups can significantly impact the efficiency of these enzymatic reactions and the resulting product profile [, , ].
Q9: Can this compound be chemically synthesized?
A9: Yes, this compound has been successfully synthesized in the laboratory using various synthetic strategies. These often involve a convergent approach, assembling smaller fragments containing specific stereochemical features of the final molecule [, , , ].
Q10: What is the significance of the C3, C5, and C11 positions on the this compound macrolactone ring for glycosylation?
A10: These positions bear hydroxyl groups that can be selectively targeted for glycosylation with different sugar moieties. Controlling the regioselectivity of glycosylation at these positions is crucial for generating desired erythromycin derivatives with specific biological activities [].
Q11: How do structural modifications to this compound impact the biological activity of erythromycin derivatives?
A11: Modifications to the this compound core, particularly changes in stereochemistry, glycosylation patterns, or the introduction of new functional groups, can significantly affect the antibacterial activity, potency, and selectivity of erythromycin derivatives. Understanding these structure-activity relationships is crucial for designing new and improved antibiotics [, ].
Q12: What are the potential future directions for research on this compound?
A12:
- Engineering EryF for Enhanced Erythromycin Production: Optimizing the activity and substrate specificity of EryF through protein engineering could lead to improved yields of erythromycin and its derivatives in heterologous expression systems [].
- Expanding the Repertoire of Hybrid Macrolides: Further exploration of glycosyltransferases from diverse bacterial sources could allow for the generation of a wider array of hybrid macrolides with novel sugar combinations, expanding the possibilities for discovering new antibiotic candidates [].
- Understanding Resistance Mechanisms: Investigating the structural basis for erythromycin resistance and how modifications to this compound affect these mechanisms is crucial for developing strategies to overcome antibiotic resistance [].
Disclaimer and Information on In-Vitro Research Products
Please be aware that all articles and product information presented on BenchChem are intended solely for informational purposes. The products available for purchase on BenchChem are specifically designed for in-vitro studies, which are conducted outside of living organisms. In-vitro studies, derived from the Latin term "in glass," involve experiments performed in controlled laboratory settings using cells or tissues. It is important to note that these products are not categorized as medicines or drugs, and they have not received approval from the FDA for the prevention, treatment, or cure of any medical condition, ailment, or disease. We must emphasize that any form of bodily introduction of these products into humans or animals is strictly prohibited by law. It is essential to adhere to these guidelines to ensure compliance with legal and ethical standards in research and experimentation.