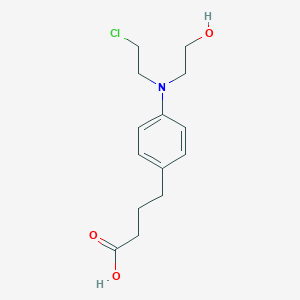
Hydroxy chlorambucil
Overview
Description
Chlorambucil is a chemotherapy agent used in the management of chronic lymphocytic leukemia and malignant lymphomas . It is a nitrogen mustard alkylating agent used as an antineoplastic agent for the treatment of various malignant and nonmalignant diseases . Although it is less toxic than most other nitrogen mustards, it has been listed as a known carcinogen .
Synthesis Analysis
While specific synthesis details for Hydroxy Chlorambucil were not found, a study describes the chemical synthesis of a new DO3A conjugate of chlorambucil as a magnetic resonance imaging (MRI) theranostic agent .
Molecular Structure Analysis
Chlorambucil is bound at the H-site attached to the thiol group of GSH, is partially ordered and exposed to the solvent, making specific interactions with the enzyme . The molecular formula of Hydroxy Chlorambucil is C14H20ClNO3 .
Chemical Reactions Analysis
While specific chemical reactions involving Hydroxy Chlorambucil were not found, a study discusses the reactions of hydroxyl radicals with benzoic acid and benzoate .
Physical And Chemical Properties Analysis
The physical and chemical properties of Chlorambucil include a molecular weight of 304.21 g/mol, melting point of 64°C to 66°C, water solubility of 12.4 g/L at 25°C, and vapor pressure of 5.7 × 10–8 mm Hg at 25°C .
Scientific Research Applications
Subheading Hydroxychloroquine Use and Ethical Considerations During the COVID-19 Pandemic
Hydroxychloroquine (HCQ), along with Chloroquine (CQ), garnered significant attention during the COVID-19 pandemic. The off-label use of these drugs necessitated a thorough ethical review to ensure people's health and safety. This review encompassed adherence to ethical standards, efficiency in review processes, safeguarding participant rights, and maintaining independent ethics committee reviews. The core principle was to maximize benefits while standardizing application and review processes to mitigate unnecessary risks (Li et al., 2022).
Bendamustine: A Relative of Chlorambucil
Subheading Insights into Bendamustine's Mechanisms and Potential for Combination Therapies
Bendamustine, bearing a chemical relation to Chlorambucil, exhibits a complex mechanism involving alkylating and antimetabolic properties. Initially designed for low-toxicity antineoplastic effects, it predominantly acts as an alkylating agent at higher concentrations. Insights into its multi-faceted action and cell cycle effects pave the way for combination therapy strategies, potentially enhancing therapeutic outcomes (Gandhi, 2002).
Metabolism and Interaction Studies
Subheading Exploring Chlorambucil's Metabolism and DNA Interaction
Chlorambucil's clinical efficacy against cancer faces challenges due to drug-resistant tumor cells. A study investigating its metabolism by rat liver microsomal glutathione S-transferase sheds light on its potential role in acquired drug resistance. The research elucidates the interactions between Chlorambucil and DNA, revealing insights into binding modes and thermodynamic parameters. This knowledge is crucial in understanding drug resistance mechanisms and improving treatment strategies (Zhang, Ye, & Lou, 2004).
Subheading Innovative Drug Delivery and Hydrolytic Stability
Recent advancements in drug delivery systems have shown promising results in enhancing the hydrolytic stability and release properties of Chlorambucil. Mesoporous polymeric microspheres have demonstrated their ability to stabilize Chlorambucil against hydrolysis, offering a sustained release mechanism that may revolutionize oral administration, especially in metronomic chemotherapy (Wang, Cao, & Yan, 2018).
Mechanism of Action
Target of Action
Hydroxy chlorambucil, like its parent compound chlorambucil, primarily targets the DNA within cells . It is an alkylating agent, which means it can add alkyl groups to many electronegative groups under conditions present in cells .
Mode of Action
Hydroxy chlorambucil interferes with DNA replication and RNA transcription by alkylation and cross-linking the strands of DNA . This cross-linking of guanine bases in DNA double-helix strands directly attacks DNA, making the strands unable to uncoil and separate . As this uncoiling and separation is necessary for DNA replication, the cells can no longer divide .
Biochemical Pathways
The primary biochemical pathway affected by hydroxy chlorambucil is DNA replication. By cross-linking DNA strands, it disrupts the normal replication process . This disruption can lead to cell cycle arrest and cellular apoptosis via the accumulation of cytosolic p53 and subsequent activation of Bcl-2-associated X protein, an apoptosis promoter .
Pharmacokinetics
The pharmacokinetics of hydroxy chlorambucil are likely similar to those of chlorambucil. Chlorambucil has a distribution half-life of 0.49 hours and a terminal elimination half-life of 2.45 hours . The bioavailability of chlorambucil decreases when treatment cycles are repeated .
Result of Action
The result of hydroxy chlorambucil’s action at the molecular and cellular level is the inhibition of cell division, leading to cell death . This is particularly effective in rapidly dividing cells, such as cancer cells .
Action Environment
The action, efficacy, and stability of hydroxy chlorambucil can be influenced by various environmental factors. For instance, the presence of other medications can affect its metabolism and elimination . Additionally, factors such as the patient’s overall health, liver function, and kidney function can also impact the drug’s effectiveness and side effects .
Safety and Hazards
Future Directions
Chlorambucil is associated with challenges such as bioavailability and severe side effects on normal tissues. The incorporation of Chlorambucil-based hybrid molecules into nanocarriers, such as liposomes, dendrimers, micelles, has been suggested as a future direction . Furthermore, this review gives an update (2010 to date) on the developments of chlorambucil hybrid compounds with anticancer activity, and the structure-activity relationship (SAR), and also highlights future strategies for developing novel anticancer agents .
properties
IUPAC Name |
4-[4-[2-chloroethyl(2-hydroxyethyl)amino]phenyl]butanoic acid | |
---|---|---|
Source | PubChem | |
URL | https://pubchem.ncbi.nlm.nih.gov | |
Description | Data deposited in or computed by PubChem | |
InChI |
InChI=1S/C14H20ClNO3/c15-8-9-16(10-11-17)13-6-4-12(5-7-13)2-1-3-14(18)19/h4-7,17H,1-3,8-11H2,(H,18,19) | |
Source | PubChem | |
URL | https://pubchem.ncbi.nlm.nih.gov | |
Description | Data deposited in or computed by PubChem | |
InChI Key |
SFCORMODVLBZLW-UHFFFAOYSA-N | |
Source | PubChem | |
URL | https://pubchem.ncbi.nlm.nih.gov | |
Description | Data deposited in or computed by PubChem | |
Canonical SMILES |
C1=CC(=CC=C1CCCC(=O)O)N(CCO)CCCl | |
Source | PubChem | |
URL | https://pubchem.ncbi.nlm.nih.gov | |
Description | Data deposited in or computed by PubChem | |
Molecular Formula |
C14H20ClNO3 | |
Source | PubChem | |
URL | https://pubchem.ncbi.nlm.nih.gov | |
Description | Data deposited in or computed by PubChem | |
DSSTOX Substance ID |
DTXSID00181641 | |
Record name | Hydroxy chlorambucil | |
Source | EPA DSSTox | |
URL | https://comptox.epa.gov/dashboard/DTXSID00181641 | |
Description | DSSTox provides a high quality public chemistry resource for supporting improved predictive toxicology. | |
Molecular Weight |
285.76 g/mol | |
Source | PubChem | |
URL | https://pubchem.ncbi.nlm.nih.gov | |
Description | Data deposited in or computed by PubChem | |
Product Name |
Hydroxy chlorambucil | |
CAS RN |
27171-89-7 | |
Record name | Hydroxy chlorambucil | |
Source | ChemIDplus | |
URL | https://pubchem.ncbi.nlm.nih.gov/substance/?source=chemidplus&sourceid=0027171897 | |
Description | ChemIDplus is a free, web search system that provides access to the structure and nomenclature authority files used for the identification of chemical substances cited in National Library of Medicine (NLM) databases, including the TOXNET system. | |
Record name | NSC119101 | |
Source | DTP/NCI | |
URL | https://dtp.cancer.gov/dtpstandard/servlet/dwindex?searchtype=NSC&outputformat=html&searchlist=119101 | |
Description | The NCI Development Therapeutics Program (DTP) provides services and resources to the academic and private-sector research communities worldwide to facilitate the discovery and development of new cancer therapeutic agents. | |
Explanation | Unless otherwise indicated, all text within NCI products is free of copyright and may be reused without our permission. Credit the National Cancer Institute as the source. | |
Record name | Hydroxy chlorambucil | |
Source | EPA DSSTox | |
URL | https://comptox.epa.gov/dashboard/DTXSID00181641 | |
Description | DSSTox provides a high quality public chemistry resource for supporting improved predictive toxicology. | |
Record name | HYDROXY CHLORAMBUCIL | |
Source | FDA Global Substance Registration System (GSRS) | |
URL | https://gsrs.ncats.nih.gov/ginas/app/beta/substances/78KV9SFF2R | |
Description | The FDA Global Substance Registration System (GSRS) enables the efficient and accurate exchange of information on what substances are in regulated products. Instead of relying on names, which vary across regulatory domains, countries, and regions, the GSRS knowledge base makes it possible for substances to be defined by standardized, scientific descriptions. | |
Explanation | Unless otherwise noted, the contents of the FDA website (www.fda.gov), both text and graphics, are not copyrighted. They are in the public domain and may be republished, reprinted and otherwise used freely by anyone without the need to obtain permission from FDA. Credit to the U.S. Food and Drug Administration as the source is appreciated but not required. | |
Retrosynthesis Analysis
AI-Powered Synthesis Planning: Our tool employs the Template_relevance Pistachio, Template_relevance Bkms_metabolic, Template_relevance Pistachio_ringbreaker, Template_relevance Reaxys, Template_relevance Reaxys_biocatalysis model, leveraging a vast database of chemical reactions to predict feasible synthetic routes.
One-Step Synthesis Focus: Specifically designed for one-step synthesis, it provides concise and direct routes for your target compounds, streamlining the synthesis process.
Accurate Predictions: Utilizing the extensive PISTACHIO, BKMS_METABOLIC, PISTACHIO_RINGBREAKER, REAXYS, REAXYS_BIOCATALYSIS database, our tool offers high-accuracy predictions, reflecting the latest in chemical research and data.
Strategy Settings
Precursor scoring | Relevance Heuristic |
---|---|
Min. plausibility | 0.01 |
Model | Template_relevance |
Template Set | Pistachio/Bkms_metabolic/Pistachio_ringbreaker/Reaxys/Reaxys_biocatalysis |
Top-N result to add to graph | 6 |
Feasible Synthetic Routes
Disclaimer and Information on In-Vitro Research Products
Please be aware that all articles and product information presented on BenchChem are intended solely for informational purposes. The products available for purchase on BenchChem are specifically designed for in-vitro studies, which are conducted outside of living organisms. In-vitro studies, derived from the Latin term "in glass," involve experiments performed in controlled laboratory settings using cells or tissues. It is important to note that these products are not categorized as medicines or drugs, and they have not received approval from the FDA for the prevention, treatment, or cure of any medical condition, ailment, or disease. We must emphasize that any form of bodily introduction of these products into humans or animals is strictly prohibited by law. It is essential to adhere to these guidelines to ensure compliance with legal and ethical standards in research and experimentation.