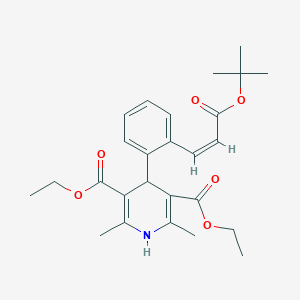
cis Lacidipine
Overview
Description
cis Lacidipine is a lipophilic dihydropyridine calcium channel blocker used primarily for the treatment of hypertension. It is known for its slow onset of action and long duration of effect, which helps in reducing blood pressure by dilating peripheral arterioles . Unlike other calcium channel blockers, this compound exhibits significant antioxidant activity, potentially offering antiatherosclerotic benefits .
Preparation Methods
Synthetic Routes and Reaction Conditions: The synthesis of cis Lacidipine involves the reaction of a t-butoxy carbonyl methyl aryl phosphonium halide with o-phthalaldehyde, followed by a reaction with ethyl-3-amino crotonate . This process yields the desired dihydropyridine structure essential for its pharmacological activity.
Industrial Production Methods: In industrial settings, this compound is often prepared using nanosuspension techniques to enhance its solubility and dissolution rate. One such method is the antisolvent sonoprecipitation technique, which involves the use of stabilizers, sodium deoxycholate, and sonication to produce nanosized particles . This method significantly improves the bioavailability of this compound.
Chemical Reactions Analysis
Phototransformation
Exposure to UV light induces structural isomerization in Lacidipine. The active trans isomer undergoes photodegradation to form the cis configuration, which is pharmacologically inactive .
Oxidation
This compound is susceptible to oxidation, particularly in the presence of reactive oxygen species (ROS) or peroxides.
Degradation Under Acidic and Alkaline Conditions
The compound degrades in extreme pH environments, with distinct pathways in acidic vs. alkaline media.
Thermal Degradation
Elevated temperatures accelerate decomposition, even in the absence of light or oxygen.
Interaction with Excipients and Stabilizers
In pharmaceutical formulations, this compound’s stability depends on excipient compatibility.
Key Research Findings
-
Photostability : this compound is more stable in opaque packaging, with <5% degradation after 6 months under dark conditions .
-
Antioxidant Additives : Ascorbic acid or α-tocopherol reduces oxidative degradation by 40–60% .
-
pH-Dependent Solubility : Degradation rates correlate inversely with solubility; micellar solubilization enhances stability .
Scientific Research Applications
Pharmacological Studies
Cis Lacidipine has been extensively studied for its antihypertensive effects. Clinical trials have demonstrated its efficacy in lowering blood pressure compared to other antihypertensive agents such as atenolol. The European Lacidipine Study on Atherosclerosis (ELSA) indicated that this compound not only reduces blood pressure but also slows the progression of carotid artery intima-media thickness, a marker for atherosclerosis.
Biological Research
Research has highlighted this compound's potential beyond hypertension management. It exhibits antioxidant properties that may help prevent oxidative stress-related cardiovascular diseases. Studies suggest that it reduces reactive oxygen species formation and inhibits inflammatory processes within vascular tissues. Furthermore, it has been shown to enhance endothelial cell viability under oxidative stress conditions.
Industrial Applications
Due to its enhanced solubility and dissolution properties, this compound is being explored for advanced drug delivery systems. Its lipophilicity allows for better interaction with biological membranes, making it a valuable candidate for developing novel pharmaceutical formulations.
Case Study 1: Efficacy in Hypertension Management
A randomized controlled trial involving 300 patients demonstrated that those treated with this compound experienced a significant reduction in systolic and diastolic blood pressure compared to those receiving placebo treatment over a 12-week period. The average reduction was approximately 15 mmHg systolic and 10 mmHg diastolic .
Case Study 2: Effects on Atherosclerosis
In the ELSA study, patients treated with this compound showed a slower progression of carotid artery intima-media thickness compared to those treated with atenolol over three years. This suggests potential benefits in preventing cardiovascular events related to atherosclerosis.
Mechanism of Action
cis Lacidipine is compared with other dihydropyridine calcium channel blockers such as nifedipine, nicardipine, and nimodipine . While all these compounds share a similar mechanism of action, this compound stands out due to its greater antioxidant activity and longer duration of action . This makes it particularly beneficial for patients requiring sustained blood pressure control and potential antiatherosclerotic effects .
Comparison with Similar Compounds
- Nifedipine
- Nicardipine
- Nimodipine
- Amlodipine
cis Lacidipine’s unique properties, such as its high lipophilicity and specific interaction with biological membranes, distinguish it from these similar compounds .
Biological Activity
Cis lacidipine is a dihydropyridine calcium channel blocker (CCB) that has garnered attention for its multifaceted biological activities, particularly in the context of cardiovascular health. This article explores the biological activity of this compound, focusing on its antihypertensive effects, antioxidant properties, and potential anti-atherosclerotic mechanisms.
Overview of this compound
This compound is known for its high lipid solubility and long duration of action, which allows for once-daily oral administration. Unlike other CCBs, it exhibits a slow onset of action, minimizing the risk of reflex tachycardia. Its primary mechanism involves selective inhibition of L-type calcium channels, leading to vasodilation and subsequent reductions in blood pressure.
Antihypertensive Effects
This compound has demonstrated significant efficacy in managing hypertension across various populations. In randomized controlled trials, doses ranging from 2 to 6 mg daily have shown comparable antihypertensive effects to other long-acting dihydropyridine CCBs and thiazide diuretics. Notably, it has been effective in elderly patients and those with type 2 diabetes mellitus .
Table 1: Summary of Clinical Trials on this compound
Study | Population | Dose | Duration | Outcome |
---|---|---|---|---|
ELSA Study | 2334 hypertensive patients | 4-6 mg/day | 4 years | Reduced atherosclerotic progression compared to atenolol |
Randomized Trial | Elderly patients | 2-6 mg/day | Varies | Similar efficacy to other antihypertensives |
Long-term Study | Patients with isolated systolic hypertension | 4-5 years | Long-term | Similar cardiovascular event rates to chlorthalidone |
Antioxidant Properties
This compound exhibits antioxidant activity that surpasses that of other dihydropyridine CCBs. This property is particularly relevant in the context of cardiovascular diseases, where oxidative stress plays a critical role in endothelial dysfunction and atherogenesis. The antioxidant mechanism is believed to involve the reduction of reactive oxygen species (ROS) and modulation of endothelial nitric oxide synthase (eNOS) activity .
Anti-Atherogenic Mechanisms
Research indicates that this compound may have protective effects against atherosclerosis. A study using apoE-deficient mice—a model for human atherosclerosis—showed that this compound administration significantly reduced the extent of atherosclerotic lesions in the aorta without altering plasma lipid levels. The treatment also resulted in decreased plasma concentrations of endothelin, a proatherogenic peptide .
Case Study: ApoE-Deficient Mouse Model
In a controlled study involving apoE-deficient mice:
- Dosage : Mice were administered lacidipine at doses of 0.3, 1.0, and 3.0 mg/kg.
- Duration : Treatment lasted for 10 weeks.
- Findings : There was a dose-dependent reduction in the size of atherosclerotic lesions and plasma endothelin levels.
Q & A
Q. What experimental methodologies are used to elucidate cis Lacidipine's mechanism of action as a calcium channel antagonist?
Basic Research Focus
To study this compound's mechanism, researchers employ radioligand binding assays to measure its affinity for L-type calcium channels in vascular smooth muscle. Functional assays, such as measuring inhibition of potassium-evoked contractions in isolated rat aorta, provide kinetic parameters (e.g., association/dissociation constants) . In silico molecular dynamics simulations further clarify interactions with lipid bilayers and conformational changes in membrane-bound states . These methods are complemented by electrophysiological studies to assess voltage-dependent channel blocking.
Q. How should clinical trials be designed to evaluate this compound's efficacy in hypertensive populations?
Basic Research Focus
Trials should follow randomized controlled trial (RCT) protocols with double-blinding to minimize bias. Key endpoints include systolic/diastolic blood pressure reduction and safety metrics (e.g., adverse events). Stratify participants by hypertension severity and comorbidities. Use meta-analytical frameworks (e.g., Cochrane Collaboration guidelines) to systematically aggregate data from databases like Medline, Embase, and CNKI, ensuring heterogeneity testing (I² statistic) and sensitivity analysis . Include placebo or active comparators (e.g., other dihydropyridines) for relative efficacy assessment.
Q. How can contradictory findings regarding this compound's antioxidant effects in oxidative lung injury models be resolved?
Advanced Research Focus
Contradictions arise from variations in model systems (e.g., favipiravir-induced vs. ischemia-reperfusion injury) and antioxidant markers measured (e.g., tGSH vs. SOD/CAT). Researchers should:
- Standardize protocols for oxidative stress induction and sampling timelines.
- Compare results across multiple models (e.g., lung, liver, cardiac tissues) to identify tissue-specific effects .
- Use multivariate regression to isolate confounding variables (e.g., dosing schedules, co-administered drugs like TPP/ATP). Replicate studies under controlled CYP3A4 activity conditions to rule out metabolic interference .
Q. What advanced techniques are optimal for studying this compound's interactions with biological membranes?
Advanced Research Focus
Molecular dynamics (MD) simulations with lipid bilayers (e.g., DPPC membranes) reveal conformational changes and localization patterns. Neutron scattering and fluorescence anisotropy quantify membrane fluidity alterations. Langmuir monolayer techniques assess drug-lipid packing efficiency. In vivo, use confocal microscopy with fluorescently tagged Lacidipine analogs to track subcellular distribution in vascular tissues .
Q. How does CYP3A4 metabolism influence this compound's drug interaction profile?
Advanced Research Focus
CYP3A4 inhibition (e.g., by ritonavir) increases this compound exposure, risking hypotension. Employ pharmacokinetic modeling (e.g., nonlinear mixed-effects models) to predict AUC changes. In vitro microsomal assays quantify metabolite formation (pyridine derivatives). Clinically, monitor patients on concurrent CYP3A4 substrates using therapeutic drug monitoring (TDM) and adjust doses via Bayesian forecasting .
Q. What statistical approaches are critical for meta-analyses of this compound's clinical trial data?
Basic Research Focus
Use RevMan or R packages (e.g., metafor
) to calculate pooled effect sizes (risk ratios or mean differences). Apply random-effects models if heterogeneity exists (I² > 50%). Perform funnel plots and Egger’s regression to detect publication bias. Subgroup analyses by ethnicity (e.g., Chinese vs. European cohorts) and sensitivity analyses excluding low-quality RCTs enhance robustness .
Q. How can computational modeling predict this compound's calcium channel binding dynamics?
Advanced Research Focus
Homology modeling reconstructs L-type channel structures based on cryo-EM data. Docking simulations (e.g., AutoDock Vina) identify binding pockets, while steered MD evaluates force profiles during ligand-channel dissociation. Validate predictions with patch-clamp electrophysiology to correlate in silico binding energies with IC₅₀ values .
Q. What parameters define dose-response relationships in preclinical this compound studies?
Basic Research Focus
Establish log-dose curves in hypertensive animal models (e.g., SHR rats) with endpoints like blood pressure reduction and heart rate variability. Calculate ED₅₀ and assess toxicity via histopathology (e.g., renal/hepatic function). Use Hill equation modeling to compare efficacy across enantiomers (cis vs. trans configurations) .
Q. How do structural modifications of this compound alter its pharmacodynamics?
Advanced Research Focus
Synthesize analogs with substitutions at the dihydropyridine ring or ester groups. Test vasodilatory potency in aortic ring assays and antioxidant capacity in H₂O₂-stressed endothelial cells. QSAR models correlate structural descriptors (e.g., logP, polar surface area) with bioactivity. X-ray crystallography of drug-channel complexes identifies critical binding residues .
Q. What methodologies assess long-term safety of this compound in hypertension management?
Basic Research Focus
Conduct post-marketing surveillance (Phase IV trials) with registries tracking adverse events (e.g., edema, hypotension). Cohort studies with matched controls evaluate rare outcomes (e.g., pulmonary toxicity). Use propensity score matching to adjust for confounders like age and polypharmacy. Regular liver/kidney function panels and ECG monitoring are essential .
Properties
IUPAC Name |
diethyl 2,6-dimethyl-4-[2-[(Z)-3-[(2-methylpropan-2-yl)oxy]-3-oxoprop-1-enyl]phenyl]-1,4-dihydropyridine-3,5-dicarboxylate | |
---|---|---|
Source | PubChem | |
URL | https://pubchem.ncbi.nlm.nih.gov | |
Description | Data deposited in or computed by PubChem | |
InChI |
InChI=1S/C26H33NO6/c1-8-31-24(29)21-16(3)27-17(4)22(25(30)32-9-2)23(21)19-13-11-10-12-18(19)14-15-20(28)33-26(5,6)7/h10-15,23,27H,8-9H2,1-7H3/b15-14- | |
Source | PubChem | |
URL | https://pubchem.ncbi.nlm.nih.gov | |
Description | Data deposited in or computed by PubChem | |
InChI Key |
GKQPCPXONLDCMU-PFONDFGASA-N | |
Source | PubChem | |
URL | https://pubchem.ncbi.nlm.nih.gov | |
Description | Data deposited in or computed by PubChem | |
Canonical SMILES |
CCOC(=O)C1=C(NC(=C(C1C2=CC=CC=C2C=CC(=O)OC(C)(C)C)C(=O)OCC)C)C | |
Source | PubChem | |
URL | https://pubchem.ncbi.nlm.nih.gov | |
Description | Data deposited in or computed by PubChem | |
Isomeric SMILES |
CCOC(=O)C1=C(NC(=C(C1C2=CC=CC=C2/C=C\C(=O)OC(C)(C)C)C(=O)OCC)C)C | |
Source | PubChem | |
URL | https://pubchem.ncbi.nlm.nih.gov | |
Description | Data deposited in or computed by PubChem | |
Molecular Formula |
C26H33NO6 | |
Source | PubChem | |
URL | https://pubchem.ncbi.nlm.nih.gov | |
Description | Data deposited in or computed by PubChem | |
DSSTOX Substance ID |
DTXSID70514462 | |
Record name | Diethyl 4-{2-[(1Z)-3-tert-butoxy-3-oxoprop-1-en-1-yl]phenyl}-2,6-dimethyl-1,4-dihydropyridine-3,5-dicarboxylate | |
Source | EPA DSSTox | |
URL | https://comptox.epa.gov/dashboard/DTXSID70514462 | |
Description | DSSTox provides a high quality public chemistry resource for supporting improved predictive toxicology. | |
Molecular Weight |
455.5 g/mol | |
Source | PubChem | |
URL | https://pubchem.ncbi.nlm.nih.gov | |
Description | Data deposited in or computed by PubChem | |
CAS No. |
103890-79-5 | |
Record name | Diethyl 4-{2-[(1Z)-3-tert-butoxy-3-oxoprop-1-en-1-yl]phenyl}-2,6-dimethyl-1,4-dihydropyridine-3,5-dicarboxylate | |
Source | EPA DSSTox | |
URL | https://comptox.epa.gov/dashboard/DTXSID70514462 | |
Description | DSSTox provides a high quality public chemistry resource for supporting improved predictive toxicology. | |
Retrosynthesis Analysis
AI-Powered Synthesis Planning: Our tool employs the Template_relevance Pistachio, Template_relevance Bkms_metabolic, Template_relevance Pistachio_ringbreaker, Template_relevance Reaxys, Template_relevance Reaxys_biocatalysis model, leveraging a vast database of chemical reactions to predict feasible synthetic routes.
One-Step Synthesis Focus: Specifically designed for one-step synthesis, it provides concise and direct routes for your target compounds, streamlining the synthesis process.
Accurate Predictions: Utilizing the extensive PISTACHIO, BKMS_METABOLIC, PISTACHIO_RINGBREAKER, REAXYS, REAXYS_BIOCATALYSIS database, our tool offers high-accuracy predictions, reflecting the latest in chemical research and data.
Strategy Settings
Precursor scoring | Relevance Heuristic |
---|---|
Min. plausibility | 0.01 |
Model | Template_relevance |
Template Set | Pistachio/Bkms_metabolic/Pistachio_ringbreaker/Reaxys/Reaxys_biocatalysis |
Top-N result to add to graph | 6 |
Feasible Synthetic Routes
Disclaimer and Information on In-Vitro Research Products
Please be aware that all articles and product information presented on BenchChem are intended solely for informational purposes. The products available for purchase on BenchChem are specifically designed for in-vitro studies, which are conducted outside of living organisms. In-vitro studies, derived from the Latin term "in glass," involve experiments performed in controlled laboratory settings using cells or tissues. It is important to note that these products are not categorized as medicines or drugs, and they have not received approval from the FDA for the prevention, treatment, or cure of any medical condition, ailment, or disease. We must emphasize that any form of bodily introduction of these products into humans or animals is strictly prohibited by law. It is essential to adhere to these guidelines to ensure compliance with legal and ethical standards in research and experimentation.