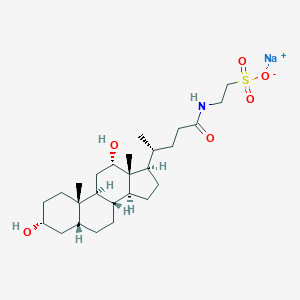
Taurodeoxycholic acid sodium salt
Overview
Description
Taurodeoxycholic acid is a bile acid, specifically a conjugate of deoxycholic acid with taurine. It is a closely related isomer of taurochenodeoxycholic acid and tauroursodeoxycholic acid, sharing the same molecular formula and molecular weight . This compound plays a significant role in the emulsification of lipids, aiding in the digestion and absorption of dietary fats.
Mechanism of Action
Target of Action
Taurodeoxycholic acid sodium salt, also known as Tauroursodeoxycholic acid (TUDCA), is a highly hydrophilic tertiary bile acid . It is a taurine conjugate of ursodeoxycholic acid . The primary targets of TUDCA are the cells in the liver and gallbladder, where it functions to regulate hydrophobic bile acids and their cytotoxic effects .
Mode of Action
TUDCA interacts with its targets by reducing the absorption of cholesterol in the small intestine, thereby reducing the body’s intake of dietary cholesterol and the body cholesterol content . It also has anti-apoptotic effects and ER stress response dampening effects . Furthermore, it has been shown to have important neuroprotective activities .
Biochemical Pathways
TUDCA affects numerous metabolic pathways, most of which lead to apoptosis .
Result of Action
The molecular and cellular effects of TUDCA’s action include the reduction of cholesterol absorption in the small intestine, regulation of hydrophobic bile acids, reduction of oxidative stress, protection of mitochondria, anti-neuroinflammatory action, and maintenance of protein stability and correct folding .
Action Environment
The action, efficacy, and stability of TUDCA can be influenced by environmental factors such as the presence of other bile acids, the pH of the environment, and the presence of lipids and proteins with which it can interact . .
Biochemical Analysis
Biochemical Properties
Taurodeoxycholic acid sodium salt plays a significant role in biochemical reactions. It is useful for the solubilization of lipids and membrane-bound proteins . It interacts with various biomolecules, including enzymes and proteins, and these interactions are crucial for its function . For instance, it has been demonstrated to stimulate chloride ion secretion through calcium-activated chloride ion channels and cystic fibrosis transmembrane conductance regulator (CFTR) in Calu-3 airway epithelial cell monolayers .
Cellular Effects
This compound exerts various effects on different types of cells and cellular processes. It influences cell function by regulating and inhibiting the apoptotic cascade, reducing oxidative stress, protecting the mitochondria, producing an anti-neuroinflammatory action, and acting as a chemical chaperone to maintain the stability and correct folding of proteins . It also has the potential to resolve endoplasmic reticulum (ER) stress in intestinal epithelial cells .
Molecular Mechanism
The molecular mechanism of action of this compound involves modulation of endoplasmic reticulum (ER) stress and mitochondrial dysfunction pathways . It exerts its effects at the molecular level through binding interactions with biomolecules, enzyme inhibition or activation, and changes in gene expression .
Dosage Effects in Animal Models
The effects of this compound vary with different dosages in animal models. It has shown important anti-apoptotic and neuroprotective activities in animal models of Alzheimer’s disease, Parkinson’s disease, Huntington’s diseases, amyotrophic lateral sclerosis (ALS), and cerebral ischemia
Preparation Methods
Synthetic Routes and Reaction Conditions: Taurodeoxycholic acid can be synthesized through the conjugation of deoxycholic acid with taurine. The reaction typically involves the activation of the carboxyl group of deoxycholic acid, followed by its reaction with taurine under controlled conditions to form the amide bond.
Industrial Production Methods: Industrial production of taurodeoxycholic acid often involves microbial fermentation processes. Engineered strains of Escherichia coli can be used to produce taurodeoxycholic acid through optimized fermentation conditions. This method is environmentally friendly and allows for large-scale production .
Types of Reactions:
Oxidation: Taurodeoxycholic acid can undergo oxidation reactions, particularly at the hydroxyl groups.
Reduction: Reduction reactions can occur, especially at the carbonyl group.
Substitution: Substitution reactions can take place at various positions on the steroid nucleus.
Common Reagents and Conditions:
Oxidizing Agents: Potassium permanganate, chromium trioxide.
Reducing Agents: Sodium borohydride, lithium aluminum hydride.
Substitution Reagents: Halogens, nucleophiles.
Major Products:
Oxidation Products: Oxidized derivatives with altered hydroxyl groups.
Reduction Products: Reduced derivatives with modified carbonyl groups.
Substitution Products: Substituted derivatives with different functional groups.
Scientific Research Applications
Taurodeoxycholic acid has a wide range of applications in scientific research:
Chemistry: Used as a reagent in various chemical reactions and analytical methods.
Biology: Plays a role in studying lipid metabolism and bile acid pathways.
Comparison with Similar Compounds
- Taurochenodeoxycholic Acid
- Tauroursodeoxycholic Acid
- Taurocholic Acid
Comparison: Taurodeoxycholic acid is unique due to its specific conjugation with taurine and its distinct molecular structure. While taurochenodeoxycholic acid and tauroursodeoxycholic acid share the same molecular formula and weight, they differ in their hydroxyl group positions and biological activities. Taurocholic acid, on the other hand, has a different bile acid conjugate and exhibits different physiological functions .
Properties
CAS No. |
1180-95-6 |
---|---|
Molecular Formula |
C26H44NNaO6S |
Molecular Weight |
521.7 g/mol |
IUPAC Name |
sodium;2-[4-[(10S,13R)-3,12-dihydroxy-10,13-dimethyl-2,3,4,5,6,7,8,9,11,12,14,15,16,17-tetradecahydro-1H-cyclopenta[a]phenanthren-17-yl]pentanoylamino]ethanesulfonate |
InChI |
InChI=1S/C26H45NO6S.Na/c1-16(4-9-24(30)27-12-13-34(31,32)33)20-7-8-21-19-6-5-17-14-18(28)10-11-25(17,2)22(19)15-23(29)26(20,21)3;/h16-23,28-29H,4-15H2,1-3H3,(H,27,30)(H,31,32,33);/q;+1/p-1/t16?,17?,18?,19?,20?,21?,22?,23?,25-,26+;/m0./s1 |
InChI Key |
YXHRQQJFKOHLAP-FONCZFQMSA-M |
Isomeric SMILES |
CC(CCC(=O)NCCS(=O)(=O)[O-])C1CCC2[C@@]1(C(CC3C2CCC4[C@@]3(CCC(C4)O)C)O)C.[Na+] |
SMILES |
CC(CCC(=O)NCCS(=O)(=O)[O-])C1CCC2C1(C(CC3C2CCC4C3(CCC(C4)O)C)O)C.[Na+] |
Canonical SMILES |
CC(CCC(=O)NCCS(=O)(=O)[O-])C1CCC2C1(C(CC3C2CCC4C3(CCC(C4)O)C)O)C.[Na+] |
melting_point |
204 - 208 °C |
1180-95-6 | |
physical_description |
Solid |
Pictograms |
Irritant |
solubility |
41 mg/mL |
Synonyms |
Acid, Taurodeoxycholic Deoxycholate, Taurine Deoxycholyltaurine Sodium Taurodeoxycholate Taurine Deoxycholate Taurodeoxycholate Taurodeoxycholate, Sodium Taurodeoxycholic Acid |
Origin of Product |
United States |
Retrosynthesis Analysis
AI-Powered Synthesis Planning: Our tool employs the Template_relevance Pistachio, Template_relevance Bkms_metabolic, Template_relevance Pistachio_ringbreaker, Template_relevance Reaxys, Template_relevance Reaxys_biocatalysis model, leveraging a vast database of chemical reactions to predict feasible synthetic routes.
One-Step Synthesis Focus: Specifically designed for one-step synthesis, it provides concise and direct routes for your target compounds, streamlining the synthesis process.
Accurate Predictions: Utilizing the extensive PISTACHIO, BKMS_METABOLIC, PISTACHIO_RINGBREAKER, REAXYS, REAXYS_BIOCATALYSIS database, our tool offers high-accuracy predictions, reflecting the latest in chemical research and data.
Strategy Settings
Precursor scoring | Relevance Heuristic |
---|---|
Min. plausibility | 0.01 |
Model | Template_relevance |
Template Set | Pistachio/Bkms_metabolic/Pistachio_ringbreaker/Reaxys/Reaxys_biocatalysis |
Top-N result to add to graph | 6 |
Feasible Synthetic Routes
Q1: Can Taurodeoxycholic acid sodium salt be used to modify graphene nanosheets for electrochemical applications?
A2: Yes, research demonstrates that this compound can functionalize graphene nanosheets. The "facial amphiphilic" structure of this compound enhances the dispersibility of the resulting composite (G-bile salts) in water. These G-bile salts can then be decorated with palladium nanoparticles to create effective electrochemical catalysts for reactions like formic acid oxidation. []
Q2: How can micellar electrokinetic capillary chromatography (MECC) be used to analyze this compound in complex mixtures?
A4: MECC proves to be a valuable technique for separating and quantifying this compound in mixtures containing other bile salts, like silymarin. By utilizing a specific buffer solution containing disodium tetraborate, this compound, and β-cyclodextrin, researchers achieved efficient separation. This method allows for accurate analysis of this compound in pharmaceutical preparations and herbal extracts. []
Q3: What is the impact of intestinal Hypoxia-inducible factor 1-alpha (HIF-1α) on bile acid profiles, including this compound, during cholestasis-induced liver injury?
A5: Research utilizing mice models with intestinal epithelial cell-specific deletion of Hif1a (Hif1aVKO) revealed a connection between intestinal HIF-1α signaling and bile acid metabolism during cholestasis. Compared to control mice, Hif1aVKO mice exhibited altered bile acid profiles in the liver, notably higher levels of this compound. These findings highlight the role of intestinal HIF-1α in regulating hepatic energy metabolism and bile acid composition during cholestasis. []
Disclaimer and Information on In-Vitro Research Products
Please be aware that all articles and product information presented on BenchChem are intended solely for informational purposes. The products available for purchase on BenchChem are specifically designed for in-vitro studies, which are conducted outside of living organisms. In-vitro studies, derived from the Latin term "in glass," involve experiments performed in controlled laboratory settings using cells or tissues. It is important to note that these products are not categorized as medicines or drugs, and they have not received approval from the FDA for the prevention, treatment, or cure of any medical condition, ailment, or disease. We must emphasize that any form of bodily introduction of these products into humans or animals is strictly prohibited by law. It is essential to adhere to these guidelines to ensure compliance with legal and ethical standards in research and experimentation.