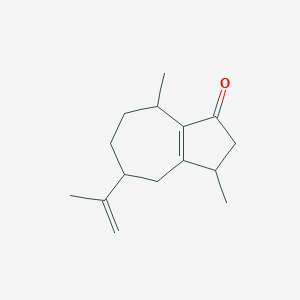
Rotundone
Overview
Description
Rotundone is a sesquiterpene originally discovered in the tubers of Java grass (Cyperus rotundus). It is also present in the essential oils of black pepper, marjoram, oregano, rosemary, basil, thyme, and geranium, as well as in some Syrah wines . It imparts a peppery aroma .
Synthesis Analysis
This compound concentration in grapes increases significantly at a time relatively close to harvest and remains then at a plateau or slightly declines .Molecular Structure Analysis
This compound has a molecular formula of C15H22O and an average mass of 218.335 Da . Its IUPAC name is (3S,5R,8S)-3,8-Dimethyl-5-(prop-1-en-2-yl)-3,4,5,6,7,8-hexahydroazulen-1(2H)-one .Chemical Reactions Analysis
This compound is the compound responsible for the “black pepper” aroma of many plant species, including several economically important wine grape varieties . Researchers now feel they understand the three mechanisms that can lead to the creation of this compound – oxidation by air, light, and enzymes .Physical And Chemical Properties Analysis
This compound is a sesquiterpene with a molecular formula of C15H22O and a molar mass of 218.340 g·mol−1 . It is colorless and practically insoluble in water but soluble in most organic solvents .Scientific Research Applications
Impact on Wine Aroma and Flavor
Rotundone is a key component influencing the aroma and flavor of wines. It is particularly significant in imparting a 'peppery' aroma to wines, making it a desirable compound in grape cultivation and wine production.
Influence of Environmental Factors on this compound Concentration : this compound levels in grape berries and wine are significantly influenced by environmental factors and seasonal conditions. Studies have shown that cooler and wetter seasons result in higher this compound concentrations in wines, contributing to the desirable peppery aroma. Additionally, the concentration of this compound in wine is negatively correlated with daily solar exposure and positively correlated with vineyard water balance (Zhang et al., 2015).
Ripeness and Viticultural Techniques : The concentration of this compound in wine is also affected by grape ripeness and viticultural practices. For instance, the degree of ripening significantly influences this compound levels, with higher concentrations reached at specific stages post-veraison. Practices such as leaf removal can reduce this compound concentration, while irrigation can increase it (Geffroy et al., 2014).
Variability Among Grape Varieties : this compound is found in many grape varieties and can be modulated using various viticultural and enological techniques. Climate change is expected to impact this compound accumulation, with changes in precipitation and temperature affecting its levels in grapes (Geffroy, Kleiber, & Jacques, 2020).
Analysis Techniques : Advanced analysis methods, like solid-phase microextraction gas chromatography/tandem mass spectrometry, have been developed for sensitive and selective routine analysis of this compound in wines. These methods have revealed the presence of this compound in unexpected concentrations in various wines, further emphasizing its role in wine aroma (Mattivi et al., 2011).
Biosynthesis and Distribution in Grapevine Tissues
Understanding the biosynthesis and distribution of this compound in grapevines is crucial for targeted cultivation strategies.
Distribution and Translocation in Grapevine Tissues : Research indicates that this compound is produced in different grapevine tissues, including leaves and stems, and its concentration varies across different developmental stages. The study also ruled out the possibility of this compound translocation between different tissues, confirming local production in individual tissues (Zhang et al., 2016).
Synthesis Methods : Innovative synthesis methods have been developed for this compound, employing biomimetic catalysts and enzymatic supply and regeneration of those catalysts. These advancements offer a platform for efficient production of this compound, which is crucial in flavor industries (Umezawa, Konishi, & Kino, 2019).
Consumer Perception and Wine Preferences
Understanding consumer preferences and perceptions regarding this compound is vital for wine marketing and production strategies.
- Consumer Preferences and Perception : Consumer response to this compound in wine is complex, with some appreciating the peppery notes while others may find them less desirable. Studies have aimed to determine consumer rejection thresholds for this compound, revealing that consumer preferences vary significantly, influenced by factors like age and specific anosmia (Geffroy et al., 2018).
Mechanism of Action
Target of Action
Rotundone is an aromatic compound found in the skin of some grapes (e.g., Shiraz, Noiret) that contributes peppery notes to wines made with these varieties . It is also found in non-grape tissues, such as leaves and stems . The primary targets of this compound are the olfactory receptors in humans that detect its characteristic “peppery” aroma .
Mode of Action
This compound has analgesic, sedative, hypnotic, and tranquilitic effects . Its analgesic effect is weaker than pethidine and stronger than general antipyretic analgesics . There is no respiratory depressive effect at therapeutic doses, nor does it cause spasm of smooth muscle of the gastrointestinal tract . While producing analgesic effects, it can cause sedation and hypnosis . The mechanism of action of this product has yet to be elucidated, which may be related to the upward activation of the system and blocking the function of dopamine receptors in the brain by inhibiting the brainstem reticular structure .
Biochemical Pathways
This compound is derived from its sesquiterpene precursor, α-guaiene, via aerial oxidation and biosynthetic transformation . The exact biochemical pathways affected by this compound and their downstream effects are still under investigation .
Pharmacokinetics
The this compound is well absorbed orally and is most distributed in the body in adipose tissue, followed by lungs, liver, and kidneys . It is excreted mainly through the kidneys . Since then, the content of internal organs has decreased, but the content of fat has increased, which is obviously related to the fat solubility of the product . Tests from mice and rabbits show that the product easily penetrates the blood-brain barrier and enters the brain tissue .
Result of Action
The molecular and cellular effects of this compound’s action primarily involve its interaction with olfactory receptors, leading to the perception of a “peppery” aroma . In addition, it has analgesic, sedative, hypnotic, and tranquilitic effects .
Action Environment
Environmental factors, particularly climatic conditions, can significantly influence the action and efficacy of this compound. For instance, vineyard growing degree days (GDD v) and the amount of solar radiation (cumulative solar exposure; CSE v) accumulated from the beginning of fruit ripening to harvest were the variables best correlated with this compound concentrations . Furthermore, this compound was negatively correlated with low (<15°C) and high (>30°C) berry temperatures . These findings suggest that the action and efficacy of this compound can be modulated by adjusting environmental and viticultural factors .
Future Directions
Biochemical Analysis
Biochemical Properties
Rotundone is produced from the precursor α-guaiene, which is synthesized by a sesquiterpene synthase, VvGuaS, from developing grape berries . The VvGuaS enzyme is a novel allele of the sesquiterpene synthase gene, VvTPS24 .
Cellular Effects
Given its potent aroma, it is likely to interact with olfactory receptors in the nose, triggering a signal transduction pathway that results in the perception of a peppery aroma .
Molecular Mechanism
The molecular mechanism of this compound involves the production of α-guaiene by the VvGuaS enzyme . Two specific polymorphisms in the VvGuaS enzyme are responsible for the production of α-guaiene .
Temporal Effects in Laboratory Settings
It is known that the concentration of this compound in grapevine tissues varies at different ripening stages .
Metabolic Pathways
This compound is involved in the terpenoid biosynthesis pathway. It is produced from the precursor α-guaiene, which is synthesized by the VvGuaS enzyme .
Transport and Distribution
It is known that the concentration of this compound in grapevine tissues varies at different ripening stages .
properties
IUPAC Name |
3,8-dimethyl-5-prop-1-en-2-yl-3,4,5,6,7,8-hexahydro-2H-azulen-1-one | |
---|---|---|
Details | Computed by Lexichem TK 2.7.0 (PubChem release 2021.05.07) | |
Source | PubChem | |
URL | https://pubchem.ncbi.nlm.nih.gov | |
Description | Data deposited in or computed by PubChem | |
InChI |
InChI=1S/C15H22O/c1-9(2)12-6-5-10(3)15-13(8-12)11(4)7-14(15)16/h10-12H,1,5-8H2,2-4H3 | |
Details | Computed by InChI 1.0.6 (PubChem release 2021.05.07) | |
Source | PubChem | |
URL | https://pubchem.ncbi.nlm.nih.gov | |
Description | Data deposited in or computed by PubChem | |
InChI Key |
NUWMTBMCSQWPDG-UHFFFAOYSA-N | |
Details | Computed by InChI 1.0.6 (PubChem release 2021.05.07) | |
Source | PubChem | |
URL | https://pubchem.ncbi.nlm.nih.gov | |
Description | Data deposited in or computed by PubChem | |
Canonical SMILES |
CC1CCC(CC2=C1C(=O)CC2C)C(=C)C | |
Details | Computed by OEChem 2.3.0 (PubChem release 2021.05.07) | |
Source | PubChem | |
URL | https://pubchem.ncbi.nlm.nih.gov | |
Description | Data deposited in or computed by PubChem | |
Molecular Formula |
C15H22O | |
Details | Computed by PubChem 2.1 (PubChem release 2021.05.07) | |
Source | PubChem | |
URL | https://pubchem.ncbi.nlm.nih.gov | |
Description | Data deposited in or computed by PubChem | |
Molecular Weight |
218.33 g/mol | |
Details | Computed by PubChem 2.1 (PubChem release 2021.05.07) | |
Source | PubChem | |
URL | https://pubchem.ncbi.nlm.nih.gov | |
Description | Data deposited in or computed by PubChem | |
CAS RN |
18374-76-0 | |
Record name | Rotundone | |
Source | Human Metabolome Database (HMDB) | |
URL | http://www.hmdb.ca/metabolites/HMDB0036443 | |
Description | The Human Metabolome Database (HMDB) is a freely available electronic database containing detailed information about small molecule metabolites found in the human body. | |
Explanation | HMDB is offered to the public as a freely available resource. Use and re-distribution of the data, in whole or in part, for commercial purposes requires explicit permission of the authors and explicit acknowledgment of the source material (HMDB) and the original publication (see the HMDB citing page). We ask that users who download significant portions of the database cite the HMDB paper in any resulting publications. | |
Retrosynthesis Analysis
AI-Powered Synthesis Planning: Our tool employs the Template_relevance Pistachio, Template_relevance Bkms_metabolic, Template_relevance Pistachio_ringbreaker, Template_relevance Reaxys, Template_relevance Reaxys_biocatalysis model, leveraging a vast database of chemical reactions to predict feasible synthetic routes.
One-Step Synthesis Focus: Specifically designed for one-step synthesis, it provides concise and direct routes for your target compounds, streamlining the synthesis process.
Accurate Predictions: Utilizing the extensive PISTACHIO, BKMS_METABOLIC, PISTACHIO_RINGBREAKER, REAXYS, REAXYS_BIOCATALYSIS database, our tool offers high-accuracy predictions, reflecting the latest in chemical research and data.
Strategy Settings
Precursor scoring | Relevance Heuristic |
---|---|
Min. plausibility | 0.01 |
Model | Template_relevance |
Template Set | Pistachio/Bkms_metabolic/Pistachio_ringbreaker/Reaxys/Reaxys_biocatalysis |
Top-N result to add to graph | 6 |
Feasible Synthetic Routes
Q & A
Q1: What is rotundone and what is it known for?
A1: this compound is a naturally occurring sesquiterpene with a distinctive peppery aroma. [] It's found in various plants, including grapes used to make wine (like Shiraz), black and white peppercorns, and even some fruits like grapefruit. [, ]
Q2: I've heard some wines are described as "peppery." Is this compound responsible for this?
A2: Yes, this compound is the primary compound responsible for the peppery aroma and flavor in wine. [, , , , ] Shiraz wines, in particular, are known for this characteristic, but it can also be found in other varieties like Duras and even some white wines. [, , , , ]
Q3: How potent is this compound's aroma?
A3: this compound has an extremely low odor detection threshold, meaning humans can perceive its peppery aroma at very low concentrations. [, ] In red wine, the threshold is around 16 ng/L, and in water, it's even lower at 8 ng/L. [, ]
Q4: Does everyone perceive the peppery aroma of this compound?
A4: Interestingly, no. Research suggests there might be a specific anosmia (inability to smell a specific scent) to this compound. [, ] Approximately 20% of people cannot detect its aroma even at high concentrations. [, ] This means the sensory experience of a peppery wine can vary drastically between individuals.
Q5: How is this compound synthesized in plants?
A5: The biosynthesis of this compound is believed to involve two major pathways: the methylerythritol phosphate (MEP) pathway and the mevalonate (MVA) pathway. [, ] These pathways lead to the production of α-guaiene, which is then oxidized to form this compound. [, ] Research suggests that the enzyme farnesyl diphosphate synthase (FPPS), involved in the MEP pathway, may play a crucial role in determining this compound accumulation in grapes. []
Q6: Do environmental factors influence this compound levels in grapes?
A6: Yes, environmental factors play a significant role in this compound accumulation. [, , , , ] Cooler growing seasons generally result in higher this compound levels in grapes and wines. [, , ] Factors like sunlight exposure, temperature, water availability, and even soil microbial communities have been linked to this compound variation within vineyards. [, , , , , ]
Q7: What is the role of sunlight in this compound production?
A7: Sunlight appears to negatively affect this compound accumulation. [, ] Studies have shown that shading grape bunches during berry development can lead to higher this compound concentrations at harvest. [, ] This suggests that the enzymatic processes involved in this compound biosynthesis might be sensitive to light.
Q8: Besides grapes, where else is this compound found naturally?
A8: this compound is present in several herbs and spices, with the highest concentration found in black and white peppercorns. [, ] It has also been detected in fruits like grapefruit, orange, apple, and mango, contributing to their complex aroma profiles. [, , ]
Q9: Can winemakers influence the peppery character of wine?
A9: Yes, certain viticultural (grape growing) and enological (winemaking) techniques can influence this compound levels and, consequently, the peppery character of wines. [, , , , , ]
Q10: What viticultural practices can impact this compound levels?
A10: Practices such as choosing specific vineyard sites with favorable microclimates, managing canopy shade to modulate berry temperature, and adjusting irrigation to influence water stress can affect this compound accumulation in grapes. [, , , , ]
Q11: How can winemakers adjust this compound during the winemaking process?
A11: While this compound is primarily extracted from grape skins, winemaking techniques that involve early skin removal, like rosé vinification, can result in lower this compound levels in the final wine. [] Conversely, extended maceration or techniques that increase skin contact might enhance peppery character, but further research is needed to fully understand these effects. [, ]
Q12: How is this compound measured in grapes and wine?
A12: Sensitive analytical techniques like gas chromatography coupled with mass spectrometry (GC-MS) are used to quantify this compound at the trace levels found in grapes and wine. [, ] These methods often involve extraction and pre-concentration steps to isolate and purify this compound before analysis.
Disclaimer and Information on In-Vitro Research Products
Please be aware that all articles and product information presented on BenchChem are intended solely for informational purposes. The products available for purchase on BenchChem are specifically designed for in-vitro studies, which are conducted outside of living organisms. In-vitro studies, derived from the Latin term "in glass," involve experiments performed in controlled laboratory settings using cells or tissues. It is important to note that these products are not categorized as medicines or drugs, and they have not received approval from the FDA for the prevention, treatment, or cure of any medical condition, ailment, or disease. We must emphasize that any form of bodily introduction of these products into humans or animals is strictly prohibited by law. It is essential to adhere to these guidelines to ensure compliance with legal and ethical standards in research and experimentation.