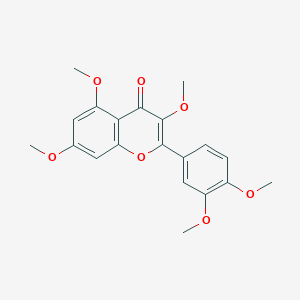
Quercetin pentamethyl ether
Overview
Description
Pentamethylquercetin is a polymethoxylated flavonoid, a derivative of quercetin, which is widely distributed in plants. This compound has garnered significant attention due to its potential therapeutic properties, including anti-carcinogenic, cardioprotective, anti-diabetic, and anti-obesity effects .
Mechanism of Action
Target of Action
Quercetin Pentamethyl Ether (QPE) is a derivative of Quercetin, a plant flavonoid found in fresh fruits, vegetables, and citrus fruits . QPE has been reported to interact directly with Sirtuin 1 (SIRT1), an NAD+ dependent deacetylase . SIRT1 is a crucial regulator that produces multiple physiological benefits, such as the prevention of cancer and age-related diseases .
Mode of Action
QPE stimulates SIRT1 activity by enhancing the binding affinity of SIRT1 with Ac-p53 peptide, a native substrate peptide . The binding affinity between SIRT1 and Ac-p53 peptide was enhanced 8.2-fold by QPE . This direct interaction and stimulation of SIRT1 activity are more effective than that of resveratrol, another well-known activator of SIRT1 .
Biochemical Pathways
The activation of SIRT1 by QPE can influence several signaling pathways within the cell. These include apoptotic, p53, NF-κB, MAPK, JAK/STAT, PI3K/AKT, and Wnt/β-catenin pathways . In addition to regulating these pathways, QPE controls the activity of oncogenic and tumor suppressor ncRNAs .
Pharmacokinetics
It is known that quercetin, from which qpe is derived, accumulates in the lungs, liver, kidneys, and small intestines, with lower levels seen in the brain, heart, and spleen . It is extracted through the renal, fecal, and respiratory systems . Due to its weak absorption through the skin, quercetin and its glycoside derivatives have been reported to be ineffective against topical inflammation, while QPE has shown potent anti-inflammatory activity with higher absorption through the skin’s surface .
Result of Action
The activation of SIRT1 by QPE can lead to various cellular and molecular effects. For instance, it can inhibit tumor proliferation, invasion, and metastasis . It can also control the production of reactive oxygen species (ROS), mitochondrial membrane depolarization, and the release of cytochrome c, demonstrating its capability to initiate apoptosis through mitochondrial pathways .
Action Environment
The action, efficacy, and stability of QPE can be influenced by various environmental factors. For instance, the environment in which the ovarian tumor develops is reported to be highly enriched with an abundance of pro-inflammatory cytokines as well as chemokines, including interleukin (IL)-1β and IL-6 and tumor necrosis factor (TNF)-α . These factors can potentially influence the action of QPE.
Biochemical Analysis
Biochemical Properties
QPE interacts with various biomolecules. For instance, it directly interacts with SIRT1, a crucial regulator that produces multiple physiological benefits . The binding affinity between SIRT1 and Ac-p53 peptide, a native substrate peptide, was enhanced 8.2-fold by QPE .
Cellular Effects
QPE has shown significant effects on various types of cells and cellular processes. For instance, it has been reported to exhibit anticardiac hypertrophy, antidiabetic, antimetabolic disorder, antithrombotic, and α-glycosidase inhibition activities .
Molecular Mechanism
QPE exerts its effects at the molecular level through various mechanisms. It enhances the binding affinity of SIRT1 with Ac-p53 peptide, a native substrate peptide without a fluorogenic moiety . This interaction stimulates SIRT1 activity .
Metabolic Pathways
QPE is involved in various metabolic pathways. The biosynthesis of quercetin, from which QPE is derived, is brought about by the phenylpropanoid metabolic pathway
Preparation Methods
Synthetic Routes and Reaction Conditions: Pentamethylquercetin can be synthesized through the methylation of quercetin. The process typically involves the use of methylating agents such as dimethyl sulfate or methyl iodide in the presence of a base like potassium carbonate. The reaction is carried out under reflux conditions to ensure complete methylation of the hydroxyl groups on the quercetin molecule .
Industrial Production Methods: Industrial production of pentamethylquercetin follows similar synthetic routes but on a larger scale. The process involves the use of continuous flow reactors to maintain consistent reaction conditions and improve yield. The purification of the final product is achieved through crystallization or chromatography techniques .
Chemical Reactions Analysis
Types of Reactions: Pentamethylquercetin undergoes various chemical reactions, including:
Oxidation: It can be oxidized to form quinones.
Reduction: Reduction reactions can convert it into dihydro derivatives.
Substitution: It can undergo nucleophilic substitution reactions, particularly at the methoxy groups.
Common Reagents and Conditions:
Oxidation: Common oxidizing agents include potassium permanganate and hydrogen peroxide.
Reduction: Reducing agents like sodium borohydride are used.
Substitution: Nucleophiles such as amines or thiols can be used under basic conditions.
Major Products:
Oxidation: Quinones and other oxidized derivatives.
Reduction: Dihydro derivatives.
Substitution: Various substituted flavonoid derivatives.
Scientific Research Applications
Chemistry: Used as a model compound to study the behavior of polymethoxylated flavonoids.
Biology: Investigated for its role in modulating adiponectin expression and improving lipid metabolism
Medicine: Potential therapeutic agent for metabolic disorders, cardiovascular diseases, and cancer
Industry: Used in the development of nutraceuticals and functional foods due to its health benefits
Comparison with Similar Compounds
Quercetin: The parent compound, widely studied for its antioxidant and anti-inflammatory properties.
Rhamnetin: Another polymethoxylated flavonoid with similar therapeutic properties.
Isorhamnetin: Known for its anti-cancer and cardioprotective effects.
Uniqueness: Pentamethylquercetin stands out due to its higher degree of methylation, which enhances its stability and bioavailability compared to other flavonoids. This makes it a more potent candidate for therapeutic applications .
Properties
IUPAC Name |
2-(3,4-dimethoxyphenyl)-3,5,7-trimethoxychromen-4-one | |
---|---|---|
Source | PubChem | |
URL | https://pubchem.ncbi.nlm.nih.gov | |
Description | Data deposited in or computed by PubChem | |
InChI |
InChI=1S/C20H20O7/c1-22-12-9-15(25-4)17-16(10-12)27-19(20(26-5)18(17)21)11-6-7-13(23-2)14(8-11)24-3/h6-10H,1-5H3 | |
Source | PubChem | |
URL | https://pubchem.ncbi.nlm.nih.gov | |
Description | Data deposited in or computed by PubChem | |
InChI Key |
ALGDHWVALRSLBT-UHFFFAOYSA-N | |
Source | PubChem | |
URL | https://pubchem.ncbi.nlm.nih.gov | |
Description | Data deposited in or computed by PubChem | |
Canonical SMILES |
COC1=C(C=C(C=C1)C2=C(C(=O)C3=C(O2)C=C(C=C3OC)OC)OC)OC | |
Source | PubChem | |
URL | https://pubchem.ncbi.nlm.nih.gov | |
Description | Data deposited in or computed by PubChem | |
Molecular Formula |
C20H20O7 | |
Source | PubChem | |
URL | https://pubchem.ncbi.nlm.nih.gov | |
Description | Data deposited in or computed by PubChem | |
DSSTOX Substance ID |
DTXSID10154467 | |
Record name | Pentamethoxyquercetin | |
Source | EPA DSSTox | |
URL | https://comptox.epa.gov/dashboard/DTXSID10154467 | |
Description | DSSTox provides a high quality public chemistry resource for supporting improved predictive toxicology. | |
Molecular Weight |
372.4 g/mol | |
Source | PubChem | |
URL | https://pubchem.ncbi.nlm.nih.gov | |
Description | Data deposited in or computed by PubChem | |
CAS No. |
1247-97-8 | |
Record name | 3,5,7,3′,4′-Pentamethoxyflavone | |
Source | CAS Common Chemistry | |
URL | https://commonchemistry.cas.org/detail?cas_rn=1247-97-8 | |
Description | CAS Common Chemistry is an open community resource for accessing chemical information. Nearly 500,000 chemical substances from CAS REGISTRY cover areas of community interest, including common and frequently regulated chemicals, and those relevant to high school and undergraduate chemistry classes. This chemical information, curated by our expert scientists, is provided in alignment with our mission as a division of the American Chemical Society. | |
Explanation | The data from CAS Common Chemistry is provided under a CC-BY-NC 4.0 license, unless otherwise stated. | |
Record name | Pentamethoxyquercetin | |
Source | ChemIDplus | |
URL | https://pubchem.ncbi.nlm.nih.gov/substance/?source=chemidplus&sourceid=0001247978 | |
Description | ChemIDplus is a free, web search system that provides access to the structure and nomenclature authority files used for the identification of chemical substances cited in National Library of Medicine (NLM) databases, including the TOXNET system. | |
Record name | Quercetin pentamethyl ether | |
Source | DTP/NCI | |
URL | https://dtp.cancer.gov/dtpstandard/servlet/dwindex?searchtype=NSC&outputformat=html&searchlist=115922 | |
Description | The NCI Development Therapeutics Program (DTP) provides services and resources to the academic and private-sector research communities worldwide to facilitate the discovery and development of new cancer therapeutic agents. | |
Explanation | Unless otherwise indicated, all text within NCI products is free of copyright and may be reused without our permission. Credit the National Cancer Institute as the source. | |
Record name | Pentamethoxyquercetin | |
Source | EPA DSSTox | |
URL | https://comptox.epa.gov/dashboard/DTXSID10154467 | |
Description | DSSTox provides a high quality public chemistry resource for supporting improved predictive toxicology. | |
Record name | PENTAMETHYLQUERCETIN | |
Source | FDA Global Substance Registration System (GSRS) | |
URL | https://gsrs.ncats.nih.gov/ginas/app/beta/substances/V6J41K636O | |
Description | The FDA Global Substance Registration System (GSRS) enables the efficient and accurate exchange of information on what substances are in regulated products. Instead of relying on names, which vary across regulatory domains, countries, and regions, the GSRS knowledge base makes it possible for substances to be defined by standardized, scientific descriptions. | |
Explanation | Unless otherwise noted, the contents of the FDA website (www.fda.gov), both text and graphics, are not copyrighted. They are in the public domain and may be republished, reprinted and otherwise used freely by anyone without the need to obtain permission from FDA. Credit to the U.S. Food and Drug Administration as the source is appreciated but not required. | |
Retrosynthesis Analysis
AI-Powered Synthesis Planning: Our tool employs the Template_relevance Pistachio, Template_relevance Bkms_metabolic, Template_relevance Pistachio_ringbreaker, Template_relevance Reaxys, Template_relevance Reaxys_biocatalysis model, leveraging a vast database of chemical reactions to predict feasible synthetic routes.
One-Step Synthesis Focus: Specifically designed for one-step synthesis, it provides concise and direct routes for your target compounds, streamlining the synthesis process.
Accurate Predictions: Utilizing the extensive PISTACHIO, BKMS_METABOLIC, PISTACHIO_RINGBREAKER, REAXYS, REAXYS_BIOCATALYSIS database, our tool offers high-accuracy predictions, reflecting the latest in chemical research and data.
Strategy Settings
Precursor scoring | Relevance Heuristic |
---|---|
Min. plausibility | 0.01 |
Model | Template_relevance |
Template Set | Pistachio/Bkms_metabolic/Pistachio_ringbreaker/Reaxys/Reaxys_biocatalysis |
Top-N result to add to graph | 6 |
Feasible Synthetic Routes
Q1: Does the choice of methylation reagent affect the outcome of QPE synthesis?
A1: Yes, using methyl iodide instead of dimethyl sulfate in the synthesis of QPE can lead to the formation of a C-methylation product, 6-methylquercetin pentamethyl ether, alongside QPE. []
Q2: Has QPE shown any potential in cancer research?
A2: Research suggests that QPE may possess anti-carcinogenic properties. In a study on mice, QPE demonstrated approximately 50% inhibition of pulmonary adenoma formation induced by benzo(a)pyrene (BP). This inhibition is linked to QPE's intermediate potency in inducing BP hydroxylase activity. Notably, β-naphthoflavone, a more potent inducer, exhibited almost total inhibition, while rutin, a weak inducer, showed no inhibition. []
Q3: Does methylation of Quercetin impact its biological activity?
A3: Yes, methylation can significantly alter the pharmacokinetic and biochemical properties of flavonoids like Quercetin. Partial methylation, in particular, can enhance metabolic stability, membrane transport, absorption, and oral bioavailability. [] For instance, Azaleatin (quercetin-5-O-methyl ether) exhibits comparable α-amylase inhibitory activity to Quercetin itself. []
Q4: What is known about the photochemical behavior of QPE?
A4: While specific details require further exploration, research indicates that QPE undergoes photooxidative cyclization. [, ] Further investigations have delved into the photochemistry of QPE, although detailed findings are not provided in the abstracts. []
Q5: Where has QPE been identified in nature?
A5: Interestingly, QPE has been isolated from the leaves of Vitex trifolia (family: Lamiaceae). This discovery is considered unusual, as typical phytochemicals from Vitex species often include terpenoids and alkaloids. []
Q6: Are there computational studies investigating the activity of QPE or its derivatives?
A6: Yes, in silico molecular modelling studies have been performed on Azaleatin, a partial methyl ether of Quercetin. These studies revealed that Azaleatin exhibits a good docking energy (-8.8 Kcalmol-1) with the α-amylase receptor. [] This suggests that Azaleatin could be a promising candidate for further structural modifications to improve its activity.
Disclaimer and Information on In-Vitro Research Products
Please be aware that all articles and product information presented on BenchChem are intended solely for informational purposes. The products available for purchase on BenchChem are specifically designed for in-vitro studies, which are conducted outside of living organisms. In-vitro studies, derived from the Latin term "in glass," involve experiments performed in controlled laboratory settings using cells or tissues. It is important to note that these products are not categorized as medicines or drugs, and they have not received approval from the FDA for the prevention, treatment, or cure of any medical condition, ailment, or disease. We must emphasize that any form of bodily introduction of these products into humans or animals is strictly prohibited by law. It is essential to adhere to these guidelines to ensure compliance with legal and ethical standards in research and experimentation.