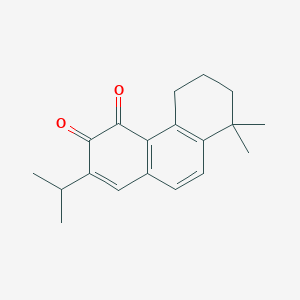
Miltirone
Overview
Description
Mechanism of Action
- Miltirone primarily interacts with the benzodiazepine receptor . Specifically, it acts as a central benzodiazepine receptor partial agonist . The benzodiazepine receptor is involved in modulating neurotransmission and plays a crucial role in anxiety, sleep regulation, and muscle relaxation.
- The primary consequence of this compound’s interaction with the receptor is an increase in intracellular calcium (Ca++) levels . This elevation in Ca++ has several effects:
- This compound’s effects are mediated through the cyclic adenosine monophosphate (cAMP) pathway . By inhibiting phosphodiesterase III (PDE-III), it prevents cAMP breakdown, leading to increased intracellular cAMP levels. This cascade ultimately results in the observed pharmacological effects .
Target of Action
Mode of Action
Biochemical Pathways
Pharmacokinetics
Result of Action
Biochemical Analysis
Biochemical Properties
Miltirone interacts with various enzymes and proteins in biochemical reactions. For instance, it has been found to interact with the phosphatidylinositol 3-kinase (PI3K)/protein kinase B (Akt) pathway . This interaction plays a crucial role in its biochemical properties .
Cellular Effects
This compound has significant effects on various types of cells and cellular processes. It has been found to attenuate reactive oxygen species (ROS)-dependent neuronal apoptosis in a cell model of Parkinson’s disease . This suggests that this compound can influence cell function, including impacts on cell signaling pathways, gene expression, and cellular metabolism .
Molecular Mechanism
This compound exerts its effects at the molecular level through several mechanisms. It has been found to activate the PI3K/Akt pathway , which is known to play a crucial role in cell survival and growth. This activation leads to a decrease in ROS generation and apoptosis in cells .
Temporal Effects in Laboratory Settings
In laboratory settings, this compound has been observed to cause a significant decrease in cell viability and increase apoptosis as early as 6 hours . These effects become more apparent after 9 hours of exposure .
Metabolic Pathways
This compound is involved in several metabolic pathways. It has been found to interact with the PI3K/Akt pathway , which is a critical regulator of cell metabolism. This interaction can lead to changes in metabolic flux or metabolite levels .
Preparation Methods
Chemical Reactions Analysis
Miltirone undergoes various chemical reactions, including:
Oxidation: this compound can be oxidized to form different quinone derivatives.
Reduction: It can be reduced to form hydroquinone derivatives.
Substitution: this compound can undergo substitution reactions, particularly at the phenanthrene ring. Common reagents used in these reactions include oxidizing agents like Dess-Martin periodinane and reducing agents like sodium borohydride. .
Scientific Research Applications
Miltirone has a wide range of scientific research applications:
Chemistry: It is used as a model compound for studying quinone chemistry and its reactivity.
Medicine: It has demonstrated neuroprotective effects in models of Parkinson’s disease by attenuating reactive oxygen species-dependent neuronal apoptosis through the PI3K/Akt pathway.
Industry: This compound’s antioxidant properties make it a valuable compound in the development of health supplements and pharmaceuticals
Comparison with Similar Compounds
Miltirone is unique among similar compounds due to its diverse pharmacological activities. Similar compounds include:
Tanshinone I: Another quinone compound from Salvia miltiorrhiza with anticancer properties.
Tanshinone IIA: Known for its cardiovascular protective effects.
Cryptotanshinone: Exhibits anti-inflammatory and anticancer activities. Compared to these compounds, this compound’s ability to modulate multiple signaling pathways and its broad range of biological activities make it a particularly versatile and valuable compound in scientific research
Properties
IUPAC Name |
8,8-dimethyl-2-propan-2-yl-6,7-dihydro-5H-phenanthrene-3,4-dione | |
---|---|---|
Source | PubChem | |
URL | https://pubchem.ncbi.nlm.nih.gov | |
Description | Data deposited in or computed by PubChem | |
InChI |
InChI=1S/C19H22O2/c1-11(2)14-10-12-7-8-15-13(6-5-9-19(15,3)4)16(12)18(21)17(14)20/h7-8,10-11H,5-6,9H2,1-4H3 | |
Source | PubChem | |
URL | https://pubchem.ncbi.nlm.nih.gov | |
Description | Data deposited in or computed by PubChem | |
InChI Key |
FEFAIBOZOKSLJR-UHFFFAOYSA-N | |
Source | PubChem | |
URL | https://pubchem.ncbi.nlm.nih.gov | |
Description | Data deposited in or computed by PubChem | |
Canonical SMILES |
CC(C)C1=CC2=C(C3=C(C=C2)C(CCC3)(C)C)C(=O)C1=O | |
Source | PubChem | |
URL | https://pubchem.ncbi.nlm.nih.gov | |
Description | Data deposited in or computed by PubChem | |
Molecular Formula |
C19H22O2 | |
Source | PubChem | |
URL | https://pubchem.ncbi.nlm.nih.gov | |
Description | Data deposited in or computed by PubChem | |
DSSTOX Substance ID |
DTXSID20181683 | |
Record name | Miltirone | |
Source | EPA DSSTox | |
URL | https://comptox.epa.gov/dashboard/DTXSID20181683 | |
Description | DSSTox provides a high quality public chemistry resource for supporting improved predictive toxicology. | |
Molecular Weight |
282.4 g/mol | |
Source | PubChem | |
URL | https://pubchem.ncbi.nlm.nih.gov | |
Description | Data deposited in or computed by PubChem | |
Physical Description |
Solid | |
Record name | Miltirone | |
Source | Human Metabolome Database (HMDB) | |
URL | http://www.hmdb.ca/metabolites/HMDB0035080 | |
Description | The Human Metabolome Database (HMDB) is a freely available electronic database containing detailed information about small molecule metabolites found in the human body. | |
Explanation | HMDB is offered to the public as a freely available resource. Use and re-distribution of the data, in whole or in part, for commercial purposes requires explicit permission of the authors and explicit acknowledgment of the source material (HMDB) and the original publication (see the HMDB citing page). We ask that users who download significant portions of the database cite the HMDB paper in any resulting publications. | |
Boiling Point |
421.00 to 422.00 °C. @ 760.00 mm Hg | |
Record name | Miltirone | |
Source | Human Metabolome Database (HMDB) | |
URL | http://www.hmdb.ca/metabolites/HMDB0035080 | |
Description | The Human Metabolome Database (HMDB) is a freely available electronic database containing detailed information about small molecule metabolites found in the human body. | |
Explanation | HMDB is offered to the public as a freely available resource. Use and re-distribution of the data, in whole or in part, for commercial purposes requires explicit permission of the authors and explicit acknowledgment of the source material (HMDB) and the original publication (see the HMDB citing page). We ask that users who download significant portions of the database cite the HMDB paper in any resulting publications. | |
CAS No. |
27210-57-7 | |
Record name | Miltirone | |
Source | CAS Common Chemistry | |
URL | https://commonchemistry.cas.org/detail?cas_rn=27210-57-7 | |
Description | CAS Common Chemistry is an open community resource for accessing chemical information. Nearly 500,000 chemical substances from CAS REGISTRY cover areas of community interest, including common and frequently regulated chemicals, and those relevant to high school and undergraduate chemistry classes. This chemical information, curated by our expert scientists, is provided in alignment with our mission as a division of the American Chemical Society. | |
Explanation | The data from CAS Common Chemistry is provided under a CC-BY-NC 4.0 license, unless otherwise stated. | |
Record name | Miltirone | |
Source | ChemIDplus | |
URL | https://pubchem.ncbi.nlm.nih.gov/substance/?source=chemidplus&sourceid=0027210577 | |
Description | ChemIDplus is a free, web search system that provides access to the structure and nomenclature authority files used for the identification of chemical substances cited in National Library of Medicine (NLM) databases, including the TOXNET system. | |
Record name | Miltirone | |
Source | EPA DSSTox | |
URL | https://comptox.epa.gov/dashboard/DTXSID20181683 | |
Description | DSSTox provides a high quality public chemistry resource for supporting improved predictive toxicology. | |
Record name | Miltirone | |
Source | Human Metabolome Database (HMDB) | |
URL | http://www.hmdb.ca/metabolites/HMDB0035080 | |
Description | The Human Metabolome Database (HMDB) is a freely available electronic database containing detailed information about small molecule metabolites found in the human body. | |
Explanation | HMDB is offered to the public as a freely available resource. Use and re-distribution of the data, in whole or in part, for commercial purposes requires explicit permission of the authors and explicit acknowledgment of the source material (HMDB) and the original publication (see the HMDB citing page). We ask that users who download significant portions of the database cite the HMDB paper in any resulting publications. | |
Melting Point |
100 °C | |
Record name | Miltirone | |
Source | Human Metabolome Database (HMDB) | |
URL | http://www.hmdb.ca/metabolites/HMDB0035080 | |
Description | The Human Metabolome Database (HMDB) is a freely available electronic database containing detailed information about small molecule metabolites found in the human body. | |
Explanation | HMDB is offered to the public as a freely available resource. Use and re-distribution of the data, in whole or in part, for commercial purposes requires explicit permission of the authors and explicit acknowledgment of the source material (HMDB) and the original publication (see the HMDB citing page). We ask that users who download significant portions of the database cite the HMDB paper in any resulting publications. | |
Retrosynthesis Analysis
AI-Powered Synthesis Planning: Our tool employs the Template_relevance Pistachio, Template_relevance Bkms_metabolic, Template_relevance Pistachio_ringbreaker, Template_relevance Reaxys, Template_relevance Reaxys_biocatalysis model, leveraging a vast database of chemical reactions to predict feasible synthetic routes.
One-Step Synthesis Focus: Specifically designed for one-step synthesis, it provides concise and direct routes for your target compounds, streamlining the synthesis process.
Accurate Predictions: Utilizing the extensive PISTACHIO, BKMS_METABOLIC, PISTACHIO_RINGBREAKER, REAXYS, REAXYS_BIOCATALYSIS database, our tool offers high-accuracy predictions, reflecting the latest in chemical research and data.
Strategy Settings
Precursor scoring | Relevance Heuristic |
---|---|
Min. plausibility | 0.01 |
Model | Template_relevance |
Template Set | Pistachio/Bkms_metabolic/Pistachio_ringbreaker/Reaxys/Reaxys_biocatalysis |
Top-N result to add to graph | 6 |
Feasible Synthetic Routes
Disclaimer and Information on In-Vitro Research Products
Please be aware that all articles and product information presented on BenchChem are intended solely for informational purposes. The products available for purchase on BenchChem are specifically designed for in-vitro studies, which are conducted outside of living organisms. In-vitro studies, derived from the Latin term "in glass," involve experiments performed in controlled laboratory settings using cells or tissues. It is important to note that these products are not categorized as medicines or drugs, and they have not received approval from the FDA for the prevention, treatment, or cure of any medical condition, ailment, or disease. We must emphasize that any form of bodily introduction of these products into humans or animals is strictly prohibited by law. It is essential to adhere to these guidelines to ensure compliance with legal and ethical standards in research and experimentation.